Artículo de revisión
Biotecnología Vegetal Vol. 20, No. 3: 157 - 166, julio - septiembre, 2020
Instituto de Biotecnología de las Plantas. UCLV. MES.
eISSN 2074-8647, RNPS: 2154
Plant growth promoting rhizobacteria: potential for sustainable agriculture
Rizobacterias promotoras del crecimiento vegetal: potencial para la agricultura sostenible
Vikas Sharma1* https://orcid.org/0000-0003-1781-1056
Jashandeep Kaur2
Shivika Sharma2
1Dept of Molecular Biology & Genetic Engg, School of Bioengineering and Biosciences. LPU-Jalandhar. India.
2Dept of Biotechnology, DAV University. Jalandhar. India.
*Author for correspondence email: biotech_vikas@rediffmail.com
ABSTRACT
With growing world population, there is an exponential increase in demand for food. The use of chemical fertilizers to improve crop productivity is associated with considerable health risks, environmental hazards, and costs. In this scenario, biofertilizers or microbial fertilizers appear to be an important alternative strategy. Therefore, this manuscript was proposed document the importance of biofertilizers and plant growth promoting rhizobacteria (PGPR) as candidates for it production. The various underlying mechanisms promoting the growth of plants, different types and comparison with chemical fertilizers are compiled in this review and the potential for developing a sustainable agriculture.
Keywords: biocontrol, biofertilizers, chemical fertilizers, nitrogen fixation, PGPRs
RESUMEN
Con la creciente población mundial, hay un aumento exponencial de la demanda de alimentos. El uso de fertilizantes químicos para mejorar la productividad de los cultivos está asociado con considerables riesgos para la salud, peligros ambientales y costos. En este escenario, los biofertilizantes o fertilizantes microbianos parecen ser una estrategia alternativa importante. Por lo tanto, este manuscrito fue propuesto para documentar la importancia de los biofertilizantes y las rizobacterias promotoras del crecimiento vegetal (PGPR) como candidatos para su producción. En esta revisión se compilan los diversos mecanismos subyacentes que promueven el crecimiento de plantas, los diferentes tipos y la comparación con los fertilizantes químicos y el potencial para desarrollar una agricultura sostenible.
Palabras clave: biocontrol, biofertilizantes, fertilizantes químicos, fijación de nitrógeno, PGPRs
INTRODUCTION
Agriculture is the science or practice that deals with crop culture and animal rearing. It is an important source of livelihood and plays a crucial role in economic development of the country. In India, two-thirds of the population depend on agriculture directly or indirectly. It is not just source of livelihood, but a great way to life. Agriculture makes the largest contribution to income. With the population growth, the demand for agriculture increases, therefore, the demand for fertilizers rises.
Fertilizers are substances containing chemical elements or chemical mixtures (nitrates, phosphates, potassium) which improve the plant growth and provide nutrition to crops. The use of commercial chemical fertilizers and pesticides enhances the productivity and development of plant growth, kill pathogen/pest. Nevertheless, they have considerable harmful effect on ecosystem as it causes water pollution, damage/ loss to plant parts, release of greenhouse gases, soil acidification, and loss of soil fertility (Youssef and Eissa, 2014).
In this scenario, an alternative is the use of Plant Growth Promoting Rhizobacteria (PGPR) (Prathap and Ranjitha, 2015). They are recognized as one of the soil and crop management practices to achieve more sustainable agriculture as well as to improve soil fertility by reducing chemical fertilizer applications (Joshi et al., 2006). This manuscript was proposed document the importance of biofertilizers and plant growth promoting rhizobacteria (PGPR) as candidates for it production. The various underlying mechanisms promoting the growth of plants, different types and comparison with chemical fertilizers are compiled in this review and the potential for developing a sustainable agriculture.
PLANT GROWTH PROMOTING RHIZOBACTERIA
Rhizosphere is narrow zone of soil influenced by plant root system and that is rich amino acid, sugars, providing source of energy and nutrients for bacteria growth. A diverse group of microorganism populates it and colonizing this habitat are called as Rhizobacteria. Microorganisms are mutual partners associated with plant growth as they have good ability for adaptation in the environment. In addition, it have capacity to support and promote the overall plant growth leading to a crop yield. These microorganisms now are incorporated in biofertilizers and biopesticides to increase soil fertility and control plant pathogens (Schroth and Hancock, 1982; Gray and Smith, 2005; Saharan and Nehra, 2011; Prashar et al., 2013).
Kloepper and Schroth (Kloepper and Schroth, 1978) coined the term PGPRs. It is particularly used as biofertilizers and biocontrol agents to improve crop productivity as well as soil fertility (Bhattacharyya and Jha, 2012).
Due to use of huge amount of chemical fertilizers, pesticides in the agricultural crop have replenish soil nitrogen and phosphorous which results in high cost and environmental pollution. PGPRs were initially used to enhance the crop fertility by increasing the amount of available nitrogen. Later they were used as biochemical agents for reducing the harmful effect of soil-borne pathogens. Different bacterial genera exhibiting plant growth-promoting activities in various soil ecosystem to make it dynamic for nutrients and sustainable crop production (Ahmed and Khan, 2009). Some common example are Pseudomonas, Azotobacter, Bacillus, Spirillium, Microbacterium, Mesorhizobium, Flavobacterium, Achromobacter, etc. (Glick, 1995; Ma et al., 2009; Narozna et al., 2014).
Plant growth promoting rhizobacteria can be classified into two groups: extracellular plant growth promoting rhizobacteria (ePGPR) and intracellular plant growth promoting rhizobacteria (iPGPR). The ePGPRs may exist in the rhizosphere, on the rhizoplane or in the spaces between the cells of root cortex. The bacterial genera such as Agrobacterium, Azosprillium, Bacillus, Azotobacter, Pseudomonas, Serratia belongs to ePGPR while iPGPRs locates generally inside the specialized nodular structure in root cells. The iPGPRs belong to the family of rhizobiaceae- Bradyrhizobium, Mesorhizobium, Frankia and Allorhizobium (Bhattacharyya and Jha, 2012; Ahmed and Kilbert, 2014; Rathore, 2015).
MECHANISM OF PLANT GROWTH PROMOTING RHIZOBACTERIA
There are several mechanisms used by plant growth promoting rhizobacteria for enhancing plant growth and development. These can be categorized as direct and indirect mechanism. Generally, plant growth promoting rhizobacteria directly promote plant growth by their ability for nutrient supply (nitrogen, phosphorus, potassium and essential minerals) and hormone production or indirectly by decreasing inhibitory effects of pathogens on plant growth and development in the form of biocontrol agents, root colonizer and environmental protectors (Bhardwaj et al., 2014; Lugtenberg and Kamilove, 2019).
Direct Mechanism
The mechanisms that stimulate plant growth directly and differ based upon the usage of particular plant species and microbial strain are defined as direct mechanisms. PGPR inhabiting root surface increases individual ion fluxes and enhances direct mineral uptake. Important direct mechanisms are discussed in the subsequent sections.
Nitrogen fixation
Nitrogen is one of the common nutrients required for plant growth and productivity. It forms an integral part of essential biomolecules. More than 80% of nitrogen is present in atmosphere as inert gas that is unavailable to the plants. Nitrogen fixation is a process by which nitrogen in the air is converted into ammonia or related nitrogenous compound. Atmospheric nitrogen is molecular dinitrogen relatively non-reactive molecule that is metabolically useless to few microorganism. Nitrogen fixation is essential to lives because fixed inorganic are required for biosynthesis of all nitrogen containing compounds which is essential for agriculture and manufacture of fertilizer. Nitrogen fixation fixes approximately 60% available nitrogen on earth (Bockman, 1997). It is economically beneficial and environmental friendly alternative to chemical fertilizer, refer to as Biological nitrogen fixation. It occurs, generally at mild temperature by nitrogen fixing microorganism that are widely distributed in nature which changes nitrogen to amino by using a complex enzyme system known as nitrogenase (Boopathi and Rao, 1999).
Plant growth promoting rhizobacteria have the ability to fix atmospheric nitrogen and provide it to plant by two mechanisms - Symbiotic and non-symbiotic. Symbiotic nitrogen fixation involves the interaction between microbe and the plant such as Rhizobium that form symbiosis with leguminous plant and Frankia with non-leguminous plant (Zahran, 2001). Non-symbiotic nitrogen fixation involves bacteria such as cynobacteria like Anabaena, Nostoc, Azotobacter, Azosprillum, Pseudomonas, Enterobacter (Vessey, 2003; Belimov et al., 2004).
Phosphate solubilization
Phosphorus is one of the major and important macronutrients for plant growth and development that plays role in metabolic processes in plant such as photosynthesis, respiration, energy transformation and macromolecular biosynthesis (Khan et al., 2010). It is abundantly available in soil but unavailable to plants because 95%-99% phosphate present in the insoluble and in immobilized form (Pandey and Maheshwari, 2007).
Phosphate solubilizing bacteria are beneficial bacteria capable of solubilizing inorganic phosphorus from insoluble compounds. The mechanism of phosphate solubilization by PGPR is associated with the release of low molecular weight organic acids and binding through their hydroxyl and carboxyl groups to phosphate, which eventually converts the insoluble phosphates to soluble form. Phosphate solubilizing bacteria attracted the attention of agriculturists as soil inoculums to improve the plant growth and yield (Valverde and Burgos, 2006). Some phosphate solubilizing bacteria produce phosphatases that hydrolyze organic forms of phosphates compound efficiently. These bacteria are referred to as phosphobacteria such as Bacillus, Arthrobacter, Beijerinckia, Enterobacter, Erwinia (Parmar and Sindhu, 2013).
Potassium solubilization
Potassium is the third major essential nutrient and the most abundantly absorbed cation and plays vital role in growth, metabolism and development of plants. Most of potassium (90%) in the soil is present in the form of insoluble rocks and silicate minerals (Kumar and Dubey, 2012). Due to imbalance, fertilizers have led to potassium deficiency that has become one of the major constraints in crop production, especially in coarse texture soil. Plant growth promoting rhizobacteria are able to solubilize potassium rocks through production and secretion of organic acids. These soil bacteria have been reported to plays a key role in the natural potassium cycle. Potassium solubilizing rhizobacteria such as Acidothiobacillus ferroxidans, Bacillus edaphicus, Bacillus mucilaginosus, Paenibacillus sp. (Liu et al., 2012) provide uptake of potassium to plants for agricultural improvement. In recent times more genera have been identified for K solubilization. Some of them are B. mucilaginosus, B. edaphicus, B. circulans, Pseudomonas, Burkholderia, Acidithiobacillus ferrooxidans, Paenibacillus spp. Action mechanisms of potassium solubilizing bacteria includes making it available for plant by organic acids production, which is a key mechanism, directly increasing dissolution by a proton- or ligand-mediated system. Besides, indirectly by forming complex structures with reaction products and thus increasing dissolution. Therefore, their application as biofertilizer enhances growth and yield of plants reducing the usage of chemical fertilizers and support environmentally sustainable crop production (Etesami et al., 2017).
Siderophores production
Siderophores are low molecular weight compound ranging 200-2000 Da commonly referred as chelate iron that are produced by microorganisms and plants. Iron is an important micronutrient for plants because it acts as cofactor in enzymatic processes, oxygen metabolism respiration, photosynthesis, etc. (Ma, 2005). The availability of iron is limited for soil microorganism thereby it have developed specific uptake strategies such as production of siderophore. This compounds producing plant growth promoting rhizobacteria can prevent the proliferation of pathogenic microorganism by assimilation of iron around the root area. The siderophores bind with ferric ion in the rhizosphere making a complex. These increases the rate of iron supply to plants and therefore enhance the plant growth and productivity of crop. Various siderophore producing bacteria belonging to the genera Bradyrhizobium, Pseudomonas, Rhizobium, Serratia and Streptomyces (Arora et al., 2013).
Phytohormone production
One of the direct mechanisms by plant growth promoting rhizobacteria is the production of plant growth regulators or phytohormones such as auxins, ethylene, gibberellins and cytokinins (Arora et al., 2013).
Diverse bacterial species produce auxins as a part of their metabolism including indole-3-acetic acid (IAA). That compound acts as plant growth regulators in plants where it controls the enlargement of cells through interaction with other plant hormones. The presence of precursor tryptophan or peptone can achieve the production of IAA by microorganism. Auxins help the plants in cell division, cell enlargement, root initiation, increased growth rate and apical dominance (Vessey, 2003). More than 80% of soil bacteria in rhizosphere are capable of producing auxins. The bacteria belongs to the genera Pseudomonas, Xanthomonas, Rhizobium, Enterobacter, Acetobacter diazotrophicus and Bradyrhizobium japonicum have shown to produce auxins which help in stimulating plant growth (Patten and Glick, 1996).
Gibberellins are important growth hormones for regulating plant growth and often it used in agriculture, horticulture and gardening. These perform active role in germination, emergence of seedlings, leaf and stem growth, induction and growth of flowers and fruits. Similarly, the growth and yield of crop plants is promoted by PGPR through gibberellin production. However, the number of gibberellins (GA) producing PGPR is small. The plant hormone level is regulated in three different ways by PGPR. One among these is direct synthesis of GA, others include de-conjugation of glucosyl gibberellins or change of inactive gibberellins into active one (Cassán et al., 2001). Besides, it have been proposed a third independent assembled pathway relative to plants and fungi that involvement of operon whose enzymatic composition indicates that gibberellin biosynthesis operate (Morrone et al., 2009). Instead of fungi, the reported pathway was superficially similar to plants. There are reports of 136 GAs from higher plants (128 species), 28 from fungi (seven species) and four from bacteria (seven species) (Kang et al., 2014).
Ethylene is important for the plant growth and development and it have distinct effects in depending of its concentration in root tissue. Higher concentration help in defoliation and cellular processes which leads to inhibition of stem and growth of root as well as premature senescence that reduces crop performance and low yield. Plant growth promoting rhizobacteria contain some important enzymes, 1-aminocyclopropane-1-carbosyilic acid (ACC deaminase). The immediate precursor for ethylene in plants is ACC. Some studies have revealed the stress induced increased ethylene synthesis from this immediate precursor in almost all plants (Liu et al., 2015; Abiri et al., 2017) and an indicator of susceptibility toward different stresses (Glick, 2014). There are so many reports regarding the isolation of PGPR possessing ACC deaminase enzyme capable of degrading ACC to ammonia and α-ketobutyrate and reducing indigenous ethylene levels of the plants (Gamalero and Glick, 2015; Raghuwanshi and Prasad, 2018). Recent literature suggests that inoculating plants with ACC deaminase producing PGPR enhances the resistance of plants towards various biotic and abiotic stresses (Pourbabaee et al., 2016; Ravanbakhsh et al., 2017; Ghosh et al., 2018; Saikia et al., 2018; Gupta and Pandey, 2019).
Indirect mechanism
Plant growth promoting rhizobacteria benefit the plant growth by indirect mechanisms related to biocontrol agents such as antibiotic, exopolysaccharides or hydrolytic enzymes, etc.
Antibiosis
The use microbial antagonist against plant pathogens in agricultural crop is suggested as a substitute for chemical pesticides. Plant growth promoting rhizobacteria like Pseudomonas, Bacillus plays a vital role in inhibiting pathogenic microorganisms by producing antibiotic. Antibiotics is one of the powerful and biocontrol agent mechanism, as different antibiotics are produce to control the proliferation of plant pathogen. A variety of antibiotics have been identified such as Amphisin, oomysin A, phenazane, tensen, tropolone and cyclic lipopeptides produce by Pseudomonas and oligomycin A, kanosamine and xanthobaccin produced by Bacillus, Streptomyces to prevent the proliferation of plant pathogens (Compant et al., 2005; Gouda et al., 2018).
Enzyme activity
Growth enhancement through enzymatic activity is another mechanism by plant growth rhizobacteria that certain enzymes such as dehydrogenase, proteases, lipase, kitinase, beta–glucanase, phosphatases attacking pathogen by excreting cell wall hydrolysis (Hayat et al., 2010). Hydrolytic enzyme degrades virulence factors or pathogens cell wall components that act indirectly for plant growth mechanism. For instance, plant growth promoting rhizobacteria through the activity of these enzymes play role to protect plants by suppression of pathogenic fungi including Botrytis cinerea, Fusarium oxysporum and Phytophthora sp. β-1,4-N-acetyl-glucoseamine and chitin are components of fungal cell wall therefore the PGPRs producing β-1,3-glucanase- and chitinase-can control their growth (Ramadan et al., 2016; Islam et al., 2016).
Exopolysaccharides production
Exopolysaccharides are carbohydrate polymer of high molecular weight that are secreated by a wide variety on PGPR. These are important in biofilm formation, root colonization, bioremediation, gelling availability, and for maintaining cellular function. Biofilm is a complex of bacterial cells attached to different biotic and abiotic surfaces that can retain moisture and protect plant roots from various pathogens (Quarashi and Sabn, 2012). Exo polysaccharides producing PGPR such as rhizobium sp., Azotobacter sp., Bacillus, Pseudomonas, Agrobacterium sp., Xanthomonas sp. helps in increasing soil fertility and contributing to sustainable agriculture. In addition, exopolysaccharides involve in cell aggregation and their synthesis may increase the chances of survival for the bacteria under desiccation and helps in nitrogen fixation by preventing high oxygen tension.
Induced systemic resistance
Colonization of plants with some plant growth promoting bacteria such as Pseudomonas putida, Serratia marcescens and Bacillus pumilus have shown to suppress disease by inducing resistance mechanism in the plant called Induced systemic Resistance (ISR). The mechanism of ISR include growth promotion, physiological tolerance, increase in production of phytoalexins, defense enzyme, antioxidant, pathogenesis related protein and modulation of phenols antimicrobial and antioxidant properties. It does not kill or inhibit of invading pathogens directly but rather on increasing chemical or physical barrier of the host plant. PGPR elicited host defense and reducing in severity the incident of disease that is caused by pathogen. Induced resistance has two major mode of action: SAR (Systemic Acquired resistance) and ISR (induced systemic resistance). The term SAR is used to describe the salicylic acid-dependent induced resistance activated by a localized infection, whereas the term ISR used to describe induced systematic promoted by non-pathogenic rhizobacteria. SAR and ISR both act through different signaling pathway. Induction of SAR occurs through salicylic acid (SA) and ISR through jasmonic acid and ethylene signaling pathway. However, these both signaling molecules together provide better protection by inducing resistance to pathogen. The role of Salicylic acid in SAR is to activate specific sets of defense –related genes known as Pathogenesis related protein (PR’s) but ISR is not followed by PR gene. PR gene are considered to increase defensive capacity of induced resistance, some of PR’s are β1,3 glucanases and chitinase (Van Loon, 2007).
CHEMICAL FERTILIZERS AND ENVIRONMENT
Chemical fertilizers have aided farmers in increasing crop production since 1930’s. The basic purpose of fertilizer application in soil is to improve the nutrient status and quality of soil by enriching it with nutrients which it lacks. With long term use of chemical fertilizers may cause water way pollution, chemical burn to crop, increased air pollution, acidification of the soil, and minerals depletion of soil Agriculture has been promoted to a very sophisticated and mechanized farming system which has result in the production of hazardous waste. The green revolution (GR) technology up until 2000 helped increase the yield of crops per hectare from 12 to 13% of food supply. In the desire to increase agricultural yield farmers have been using chemicals such as fertilizers, pesticides, insecticides, herbicides etc. The use of chemical fertilizer on crop can have adverse effect on water ways by chemical run off the excess fertilizer (Ajmal et al., 2018).
The existing organisms living in the water use up the oxygen that is left. The result is oxygen depletion causing fish to die. Chemical fertilizers are high in nutrient content such as nitrogen. Over application of chemical fertilizers to plant may cause the leaves to turn yellow or brown damaging the plant and reducing crop field. The condition is known as chemical leaf scorch. Leaf scorch can cause the leaves of the plant to wither and may cause the plants to die. Excess nitrogen used in crop fertilization can contribute to the release of greenhouse gases, such as carbon dioxide, nitrous oxide into the atmosphere. The effect is caused by using a greater amount of fertilizer than the plant can readily absorb. The over use of chemical fertilizer can lead to soil acidification damage top soil, resulting in reduced crop yield. There is an increasing concern that continues with use of chemical fertilizer on degradation essential nutrients of soil. As result the food produced in these soils have less vitamins and minerals like magnesium, potassium, and calcium. The only one alternative for chemical fertilizers is biofertilizer which enhance crop productivity and stimulate plant growth by providing essential nutrients and at the same time being ecofriendly as discussed (Umesha et al., 2018). Some elements to compare chemical fertilizer with biofertilizer are provided in table 1.
Table 1. Comparison of chemical fertilizers and biofertilizers.
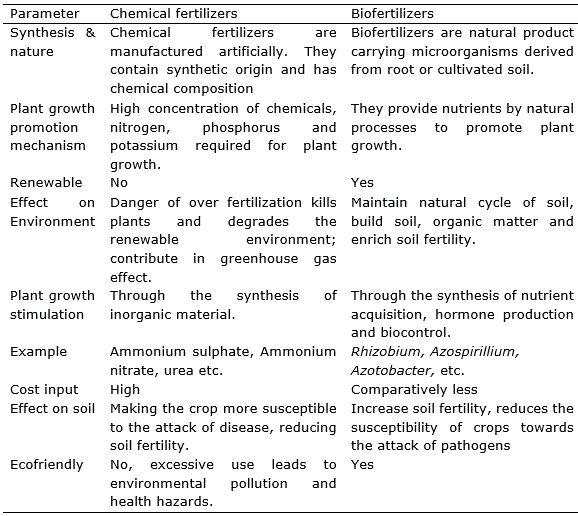
CHALLENGES AND ROLE OF BIOTECHNOLOGY IN PGPR RESEARCH
Biotechnology can play an integral role in developing biofertilizers more efficiently and on a rapid scale. There are many reports on the effective roles of different microbes using different processes to promote growth. Genetic engineering tools can play an important role in developing PGPRs with greater efficiency. However, the use of this technology is limited at this time due to associated beliefs and disbeliefs regarding the harmful or unwanted effects of the transgene or the development of pathogenicity or toxicity. However, still agricultural research can still benefit from the use of other biotechnological methods/resources to screen the beneficial plant microbial interaction. For instance, in vitro biotization is one such method that has been used to detect beneficial plant microbial interaction on a rapid scale in the potential medicinal plant Swertia chirayita Roxb. Ex Fleming (Sharma et al., 2015).
One another study has been done using plant tissue culture to see the effect of microbial products checking growth of other microbial contaminants and ensuring the growth of target plant (Sharma et al., 2018). The basic idea is to develop these methods as rapid bioassays to screen and establish positive events of plant microbial interaction and then these can be validated with the aid of molecular biology techniques. Also, the plant tissue culture can be used to screen positive microbial consortium that promote plant growth. This can be revolutionizing as it allows a rapid screening of mixed microbial population that are having symbiosis and promotion effect on plant growth, which is otherwise difficult, or time consuming. This knowledge can lead us to develop rapid and effective biofertilizers.
CONCLUSIONS
With increase in continuous human population, there is worldwide growth in food demand. However, over dependence on artificial fertilizers and pesticides have led to hazardous effects on human health, environment, and disturbance of ecological balance. Hence, PGPRs are considered a model systems which by performing various direct and indirect activities for plant growth and hence play diverse uses in agriculture and environmental sustainability. It has been proven that PGPRs can be effective and there are microbial formulations enriching the soil fertility and plant nutrition via nutrient acquisition (Nitrogen fixation, phosphate solubilization, siderophore formation, PGR production and biocontrol) and enhance agricultural yield. PGPR in terms of biofertilizer, biocontrol and bioremediation have positive influence on crop productivity and ecosystem in comparison to chemical fertilizers.
ACKNOWLEGMENT
The cooperation of Biotechnology department, DAV University is duly acknowledged.
Conflict of interest
Authors declare no conflict of interest.
Author contributions
Conceptualization VS, Formal analysis VS and JK, Resources VS and SS, Supervision VS, writing—original draft JK and VS, Writing—review & editing SS and VS.
REFERENCES
Abiri R, Shaharuddin NA, Maziah M, Yusof ZNB, Atabaki N, Sahebi M (2017) Role of ethylene and the APETALA 2/ethylene response factor superfamily in rice under various abiotic and biotic stress conditions. Environmental and Experimental Botany 134: 33-44
Ahmed M, Khan MS (2009) Effect of insecticide-tolerant and plant growth promoting Mesorhizobium on the performance of chickpea grown in insecticide stressed alluvial soils. Journal of Crop Science and Biotechnology 12: 213-222
Ahmed M, Kilbert M (2014) Mechanisms and applications of plant growth promoting rhizobacteria: current perspective. J of King Saud University Science 26: 1-20
Ajmal M, Ali HI, Saeed R, Akhtar A, Tahir M, Mehboob MZ, Ayub A (2018) Biofertilizer as an alternative for chemical fertilizers. Journal of Agriculture and Allied Sciences 7: 1-7
Arora NK, Tewari S, Singh R (2013) Multifaceted Plant-Associated Microbes and Their Mechanisms Diminish the Concept of Direct and Indirect PGPRs. In: Arora N (eds). Plant Microbe Symbiosis: Fundamentals and Advances, pp. 411-449. Springer, New Delhi; 10.1007/978-81-322-1287-4_16
Belimov AA, Kunakova AM, Safronova VI (2004) Employment of rhizobacteria for the inoculation of barley plants cultivated in soil contaminated with lead and cadmium. Microbiology 73: 99-106
Bhardwaj D, Ansari MW, Sahoo RK, Tuteja N (2014) Biofertilizers function as key player in sustainable agriculture by improving soil fertility, plant tolerance and crop productivity. Microbial Cell Factory 13: 66
Bhattacharyya PN, Jha DK (2012) Plant growth promoting rhizobacteria: emergence in agriculture. World Journal of Microbial Biotechnology 28:1327-1350
Bockman OC (1997) Fertilizers and biological nitrogen fixation as source of plant nutrients: perspectives for future agriculture. Plant soil 194: 11-14
Boopathi E, Rao KS (1999) A siderophore from Pseudomonas putida type A1: structural and biological structural and biological characterization. Biochimica et biophysica Acta (BBA)- Protein structure and molecular enzymology 1435: 30-40
Cassán F, Bottini R, Schneider G, Piccoli P (2001) Azospirillum brasilense and Azospirillum lipoferum hydrolyze conjugates of GA20 and metabolize the resultant aglycones to GA1 in seedlings of rice dwarf mutants. Plant Physiology 125: 2053-2058
Compant S, Reiter B, Sessitsch A, Nowak J, Clément C, Barka E (2005) Endophytic colonization of Vitis vinifera L. by PGPR. Applied Environmental Microbiology 71: 1685-1693
Etesami H, Emami S, Alikhani HA (2017) Potassium solubilizing bacteria (KSB): mechanisms, promotion of plant growth, and future prospects- A review. Journal of Soil Science and Plant Nutrition 17: 897-911
Gamalero E, Glick BR (2015) Bacterial modulation of plant ethylene levels. Plant Physiology 169: 13-22
Ghosh PK, De TK, Maiti TK (2018) Role of ACC Deaminase as a Stress Ameliorating Enzyme of Plant Growth-Promoting Rhizobacteria Useful in Stress Agriculture: A Review. In: Meena V (eds). Role of Rhizospheric Microbes in Soil, pp. 57-106. Springer, Singapore; doi:10.1007/978-981-10-8402-7_3
Glick BR (1995) Enhancement of plant growth by free living bacteria. Canadian Journal of Microbiology 41: 109-117
Glick BR (2014) Bacteria with ACC deaminase can promote plant growth and help to feed the world. Microbiological Research 169: 30-39
Gouda S, Kerry RG, Das G, Paramithiotis S, Shin HS, Patra JK (2018) Revitalization of plant growth promoting rhizobacteria for sustainable development in agriculture. Microbiological Research 206: 131-140
Gray EJ, Smith DI (2005) Intracellular and Extracellular PGPR: commonalities and distinctions in the plant –bacterium signaling processes. Soil Biology and Biochemistry 37: 395-412
Gupta S, Pandey S (2019) ACC Deaminase producing bacteria with multifarious plant growth promoting traits alleviates salinity stress in French bean (Phaseolus vulgaris) plants. Frontiers in Microbiology 10: 1506
Hayat R, Ali S, Ahmed I (2010) Soil beneficial bacteria and their role in PGPR. Microbiology 60: 579-598
Islam S, Akanda AM, Prova A, Islam MdT, Hossain Md(2016) Isolation and identification of plant growth promoting rhizobacteria from cucumber rhizosphere and their effect on plant growth promotion and disease suppression. Frontiers in Microbiology 6: 1-12
Joshi KK, Kumar V, Dubey RC, Maheswari DK (2006) Effect of chemical fertilizer adaptive variants, Pseudomonas aeruginosa GRC2 and Azotobacter chroococcum AC1 ON Macrophomina phaseolina causing charcoal rot Brassica juncea . Korean Journal of Environmental Agriculture 25: 228-235
Kang SM, Waqas M, Khan AL, Lee IJ (2014) Plant-growth-promoting rhizobacteria: potential candidates for gibberellins production and crop growth promotion. In: Miransari M (eds). Use of Microbes for the Alleviation of Soil Stresses, pp.1-19. Springer, New York; doi:10.1007/978-1-4614-9466-9_1
Khan MS, Zaidi A, Ahmed M, Oves M (2010) Plant growth promotion by phosphate solubilizing bacteria. Acta Microbial Immunol Hung 56: 263-284
Kloepper JW, Schroth MN (1978) Plant growth promoting rhizobacteria on radishes. Proc 4th Int Conf Plant Path Bact. INRA, Anger
Kumar P, Dubey RC (2012) PGPR for biocontrol for phythopathogens and yield in P. vulgaris. Journal of Current Perspectives in Applied Microbiology 1: 6-38
Liu D, Lian B, Dong H (2012) Isolation of Paenibacillus sp. and assessment for enhancing minerals. Geomicrobiology Journal 29: 413-421
Liu J, Xie B, Shi X, Ma J, Guo C (2015) Effects of two plant growth-promoting rhizobacteria containing 1-aminocyclopropane-1-carboxylate deaminase on oat growth in petroleum-contaminated soil. International Journal of Environmental Science and Technology 12: 3887-3894
Lugtenberg B, Kamilove F (2019) PGPR. Annual Reviews in Microbiology 63: 541-556
Ma JF (2005) Plant responses to soil minerals. Plant Sciences 24:267-281
Ma Y, Rajkumar M, Freitas H (2009) Inoculation of plant growth promoting bacteria Achromobacter xylosaxidans strains AX10 for the improvement of copper phythoextration by Brassica junicea. Journal of Environmental Management 90: 831-837
Morrone D, Chambers J, Lowry L, Kim G, Anterola A, Bender K, Peters RJ (2009) Gibberellin biosynthesis in bacteria: separate ent-copalyldiphosphate and ent-kaurene synthases in Bradyrhizobium japonicum. FEBS Letters 583: 475-480
Narozna D, Pudelko K, Kroliczak J, Golinska B, Sugawara M, Madrazak CJ, Sadowsky MJ (2014) Survival and competitiveness of Bradyzhizobium japonium strains 20 yrs after introduction into field location in Poland. Applied Environmental Microbiology 81: 5551-5559
Pandey P, Maheshwari DK (2007) Two sp. microbial contortion for growth promotion. Current Science 92: 1137-1142
Parmar P, Sindhu (2013) Potassium solubilization by rhizobacteria. Journal of Microbial Research 3: 25-31
Patten CL, Glick BR (1996) Bacterial biosynthesis of IAA. Canadian Journal of Microbiology 42: 207-220
Pourbabaee A, Bahmani E, Alikhani H, Emami S (2016) Promotion of wheat growth under salt stress by halotolerant bacteria containing ACC deaminase. JAST 18: 855-864
Prashar P, Kapoor N, Sachdeva S (2013) Rhizosphere: its structure, bacterial diversity and significance. Review in Environmental Science and Biotechnology 13: 63-67
Prathap M, Ranjitha KBD (2015) A critical review on plant growth promoting rhizobacteria. Journal of Plant Pathology and Microbiology 64: 1-4
Quarashi AW, Sabn AN (2012) Bacterial exopolysaccharide and biofilm formation in chickpea. Brazilian Journal of Microbiology 43: 1183-1191
Raghuwanshi R, Prasad JK (2018) Perspectives of rhizobacteria with ACC Deaminase activity in plant growth under abiotic stress. In: Giri B, Prasad R, Varma A (eds). Root biology, pp. 303-321. Springer, Cham
Ramadan EM, Abdelhafez AA, Hassan EA, Saber FM (2016) Plant growth promoting rhizobacteria and their potential for biocontrol of phythopathogens. African Journal of Microbial Research 10: 486-504
Rathore P (2015) A review on approaches to develop plant growth promoting rhizobacteria. Journal of Plant Physiology 176: 47-54
Ravanbakhsh M, Sasidharan R, Voesenek LA, Kowalchuk GA, and Jousset A (2017) ACC deaminase-producing rhizosphere bacteria modulate plant responses to flooding. Journal of Ecology 105: 979-986
Saharan BS, Nehra V (2011) Plant growth promoting rhizobacteria: A critical review. Life sciences Medical Research 1: 21-29
Saikia J, Sarma RK, Dhandia R, Yadav A, Bharali R, Gupta VK (2018) Alleviation of drought stress in pulse crops with ACC deaminase producing rhizobacteria isolated from acidic soil of Northeast India. Scientific Reports 8: 3560
Schroth MN, Hancock JG (1982) Disease-suppression soil and root-colonizing bacteria. Science 216: 76-81
Sharma V, Kamal B, Srivastava N, Negi Y, Dobriyal AK, Jadon VS (2015) Enhancement of in vitro growth of Swertia chirayita Roxb. Ex Fleming co-cultured with plant growth promoting rhizobacteria. Plant Cell Tissue and Organ Culture 121: 215-225
Sharma V, Kumari K, Chaudhary P, Sharma S (2018) Elicitor mediated enhanced caulogenesis and reduced contamination during micropropagation in Ocimum sanctum L. Research Journal of Biotechnology 13: 92-97
Umesha S, Singh PK, Singh RP (2018) Microbial Biotechnology and Sustainable Agriculture. In: Singh RL, Mondal S (eds). Biotechnology for Sustainable Agriculture, pp. 185-205. Woodhead Publishing, Kidlington; doi:10.1016/B978-0-12-812160-3.00006-4
Valverde A, Burgos F (2006) Effects of phosphate solubilizing bacteria on the growth of chickpea. Plant Soil 287: 43-50
Van Loon LC (2007) Plant responses to PGPR. European Journal of Plant Pathology 119: 243-254
Vessey JK (2003) PGPR as biofertilizers. Plant Soils 255: 571
Youssef MMA, Eissa MFM (2014) Biofertilizers and their role in management of plant parasitic nematodes. E3 Journal of Biotechnology and Pharmaceutical Research 5: 1-6
Zahran HH (2001) Rhizobia from wild legumes: diversity, taxonomy, ecology, nitrogen fixation and biotechnology. Journal of Biotechnology 91: 143-153
Recibido: 15-02-2020
Aceptado: 05-05-2020
Copyright (c) 2021 Biotecnología Vegetal
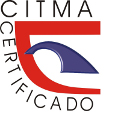
Biotecnología Vegetal eISSN 2074-8647, RNPS: 2154. ISSN 1609-1841, RNPS: 0397 Editada por: Instituto de Biotecnología de las Plantas. Universidad Central Marta Abreu de Las Villas. Carretera a Camajuaní km 5.5, Santa Clara, Villa Clara, Cuba CP 54 830 Tel: 53 42200124, e-mail: info@ibp.co.cu
Biotecnología Vegetal está bajo una Licencia Creative Commons Atribución-NoComercial 4.0 Internacional.