Reseña Científica Biotecnología Vegetal Vol. 11, No. 2: 67 - 75, abril - junio, 2011
ISSN 1609-1841 (Versión impresa)
ISSN 2074-8647 (Versión electrónica)
Oxidative burst in plant-pathogen interaction
Milady Mendoza
Instituto de Biotecnología de las Plantas, Universidad Central Marta Abreu de Las Villas. Carretera a Camajuaní km 5.5, Santa Clara, Villa Clara, Cuba. CP 54 830. e-mail: milady@ibp.co.cu
ABSTRACT
The production of reactive oxygen species (ROS) via consumption of oxygen in a so-called oxidative burst is a hallmark of successful recognition of infection and activation of plant defenses. ROS are not only toxic by-products of aerobic metabolism, but are also signalling molecules involved in several developmental processes in all organisms. Previous studies have shown that an oxidative burst often takes place at the site of attempted invasion, during the early stages of most plant-pathogen interactions. For this reason, in order to cope with the deleterious effects of these molecules, plants are fitted a large panel of enzymatic and non-enzymatic antioxidant mechanisms. Here, is presented some of the main aspects which are related with ROS role during plant-pathogen interaction.
Key words: antioxidant mechanisms, biotic stress, plant defense
RESUMEN
La producción de especies reactivas de oxígeno (ROS) por la vía de consumo de oxígeno en lo que se denomina explosión oxidativa, es un sello del reconocimiento exitoso de la infección y activación de la defensa de la planta. ROS no son solo productos tóxicos del metabolismo aeróbico, sino además, moléculas señales involucradas en varios procesos de desarrollo en todos los organismos. Estudios previos han demostrado que una explosión oxidativa frecuentemente tiene lugar en el sitio de la invasión, durante las primeras etapas de la mayoría de las interacciones planta-patógeno. Por esta razón, con vista a poder enfrentar el efecto dañino de estas moléculas, las plantas han ajustado un gran panel de mecanismos antioxidantes enzimáticos y no-enzimáticos. Aquí, se presentan algunos de los principales aspectos relacionados con el papel de ROS durante la interacción planta-patógeno.
Palabras clave: defensa de la planta, estrés biótico, mecanismos antioxidantes
CONTENTS
INTRODUCTION ROS SPECIES
The first line of defense in plants
ROS scavenging machinery in plant cells ROS FUNCTION IN PLANT DEFENSE
Roles of ROS in hostpathogen interactions
ROS in biotic interaction
ROS as molecular signaling
INTRODUCTION
Throughout their life cycle, plants have to react to various threats coming from the outside environment. That is why they have developed a broad range of strategies, collectively known as defense or stress responses, to protect themselves against abiotic (temperature, drought, etc.) and biotic (pathogenic fungi, bacteria and viruses) factors (Breusegem and Dat, 2006).
To cope with this stress response plants possess physical barriers, such as the cuticle, the cell wall and a number of biological and molecular mechanisms to counteract this effect, which includes the synthesis ROS, namely the oxidative burst. This reaction include the production at the cell surface of different molecules such as: hydrogen peroxide (H2O2), superoxide (O2-), singlet oxygen (O2) and hydroxyl radical (OH-). Specifically, against microorganisms a sophisticated sensory
system enables them to perceive chemical signals from potential pathogens and to translate them into appropriate biochemical responses (Wojtaszek, 1997; Hancock et al., 2001).
The oxidative burst has been known for more than 30 years in mammals from studies on the respiratory burst in phagocytes (Wientjes and Segal, 1995), however, in plants the phenomenon was demonstrated much later (Doke, 1983).
In biological systems oxidative stress results from the presence of elevated levels of oxidizing agents that are able to abstract electrons from essential organic molecules and disturb cellular functions. Under normal conditions ROS appear in cells as unwelcome harmful by-products formed as a result of successive one-electron reductions of molecular oxygen (Ryter et al., 2007).
As a consequence of disturbances in the normal redox state of the cell ROS molecules are produced, which have a toxic effect on it and damage all components inside them including proteins, lipids, and DNA. The magnitude of this damage depends upon the size of these changes, with a cell being able to overcome small perturbations and regain its original state. Most plant cells possess facing an even greater burden of ROS has the ability to detoxify it and have also acquired the relevant protective mechanisms to maintain the lowest possible levels of ROS inside. To these protective mechanisms belong some antioxidant molecules (á-tocopherol, ascorbate (ASC), glutathione (GSH), proline, betaine and carotenoids) and antioxidant enzymes like superoxide dismutase (SOD), ascorbate peroxidase (APX) and catalase (CAT). However, more severe oxidative stress can cause cell death and even moderate oxidation can trigger apoptosis, while more intense stresses may cause necrosis (Torres et al., 2006; Shetty et al., 2008).
It is known that one of the earliest of many diverse defense reactions activated in plan tissues in response to pathogen attack, is the rapid and transient accumulation of huge amounts of ROS and depending on the interaction, these ROS-generating
mechanisms involve plasma membrane NADPH oxidases or cell-wall peroxidases (Torres, 2010).
Studies related with the role of ROS during plant-pathogen interaction have been carried out in all kind of interactions. In hemibiotrophic interaction by (Able et al., 2000; Pignocchi et al., 2006; Shetty et al., 2007), in necrotrophic interaction by (García-Limones et al., 2002; Davies et al., 2006; Asselbergh et al., 2007; García-Limones et al., 2009) and in biotrophic interaction by (Bechtold et al., 2005; Romero et al., 2008). Besides another ones regarding to this theme have been done by (Bindschedler et al., 2006; Torres et al., 2006; Zago et al., 2006; Choi et al., 2007; Shetty et al., 2008).
In spite of this plethora of information about ROS role in different plant-pathogen interactions, knowledge is still scarcely and not enough for a complete understanding of the oxidative stress in plants although, it is believed that during an interaction a coordinated activation at the site of infection requires tight control of the production of ROS, such as H2O2 and O2-. Besides, some research have indicated that the ROS produced in the oxidative burst could serve not only as protectant against invading pathogen, but could also be the signals activating further plant defence reactions (Kim et al., 2008; Van Breusegem et al., 2008).
In the present article an overview of the main aspects related with plant-pathogen interaction is given.
ROS SPECIES
In the plant cell the term ROS is used to describe the products of the sequential reduction of molecular oxygen. They are produced at a low level in non-stressed cells in different organelles such as: chloroplasts, mitochondria, microbodies, peroxisomes, being in chloroplasts the Mehler reaction, the primary source of it. The main characteristics of the different ROS molecules are:
- H2O2, is a relatively stable ROS being not very reactive and electrically neutral, is able to pass through cell membranes and reach cell locations remote from the site of its formation. Together with O2- can be converted to
hydroxyl radicals (which are very strong oxidants) by the Haber-Weiss reaction.
- O2-, in living cells exists in equilibrium with its protonated form, the hydroperoxyl radical (O2H-). At a physiological pH is not very reactive against major macromolecular components of the cell.
- OH-, is the most reactive specie that could be formed directly through Haber-Weiss reaction. Although significant levels could be produced through the cycle of reactions that involve oxidation of transition metals such as Fe2+ and Cu+. It is believed that it is the major ROS responsible for the irreversible modifications of cellular macromolecules and damage of organelles (Wojtaszek, 1997; lesak et al., 2007).
ROS molecules have an important role in some physiological processes like plant growth and development (Laloi et al., 2004) and previous work suggested that the oxidative burst could have a direct effect on pathogen or the defences because of its activity.
ROS could directly kill the pathogen, especially in the case of the more reactive species like hydroxyl radicals which are produced in response to pathogens. These molecules also can contribute to the establishment of physical barriers at the large papillae, that are formed at the site of interaction of many pathogens by cross linking of cell wall glycoproteins or via oxidative cross linking of precursors during the localized biosynthesis of lignin and suberin polymers (Lamb and Dixon, 1997; Hückelhoven, 2007).
Evidence suggests that ROS also have a signaling function mediating defense gene activation and establishment of additional defenses, by redox control of transcription factors or by interaction with other signaling components like phosphorylation cascades. ROS can generate lipid derivatives by non-enzymatic oxygenation that can produce membrane damage or function as signaling
molecules like cyclic oxylipins of the jasmonate type. Also, can mediate the generation of phytoalexins and secondary metabolites that arrest pathogen growth (Apel and Hirt, 2004; Mittler et al., 2004; Torres et al., 2006). But, ROS are most distinctively associated with the HR, a localized response at the site of pathogen attack that displays programmed cell death and that could contribute to limit the spread of the pathogens or be a source of signals for establishment of further defenses (Narusaka et al., 2004; Mur et al., 2007).
The first line of defense in plants
In resistant plants when a pathogen is detected the first responses occur at the site of infection within minutes of invasion. These rapid events are transcription-independent, cause morphological and physiological changes in the infected cells and their surroundings and dependent on allosteric changes of several enzymes and fast chemical reactions. A massive synthesis of ROS is produced mainly in the apoplast, although it can be produce in other cellular compartments. At the same time ion fluxes, cytoskeletal rearrangements, protein phosphorylation/dephosphorylation, nitric oxide (NO) synthesis, transcriptional and post-translational activation of transcription factors takes place. Finally, a second sustained phase that occurs hours after pathogen attack usually associated with the establishment of the defenses and the hypersensitive response is carried out. All these events act as the first line of defense, slowing down the pathogens spread and initiating a signaling mechanism that leads to more fundamental changes in the metabolism of the infected plant (Gara et al., 2003; Bindschedler et al., 2006; Davies et al., 2006; Zaninotto et al., 2006; Choi et al., 2007).
ROS scavenging machinery in plant cells
Like most aerobic organisms, plants possess the ability to produce and detoxify ROS. In plants, under normal physiological conditions, ROS are produced during the process of molecular oxygen assimilation and under stress a rapid, intensive production of ROS is done. This excess leads to cellular damage and ultimately to cell death, primarily through
damage to the photosystem II reaction centre and to membrane lipids. That is why, plants have evolved an elaborate enzymatic and non-enzymatic antioxidant mechanisms to scavenge excess ROS to prevent cellular damage, including up-regulation of antioxidant defense mechanisms, such as small antioxidant molecules like á-tocopherol, ASC, GSH, proline, betaine, carotenoids and antioxidant enzymes such as SOD, APX and CAT (Shao et al., 2008).
The ROS scavenging mechanism constitute the first line of antioxidant defense in plants preventing ROS formation. Metal chelators can prevent the formation of ROS by suppressing processes such as the Haber-Weiss reaction and consequently reducing the production of the very reactive OH-.
The second line of antioxidant defense is composed of antioxidant enzymes and low molecular weight compounds. If the first line of antioxidant defense fails to prevent the formation of ROS, antioxidant components discompose reactive species avoiding the formation of oxidative lesions in biomolecules. If reactive species cannot be eliminated and succeed in attacking biomolecules, various systems are able to repair oxidative lesions produced. All these components do not act individually and there are regulatory systems, which coordinate the oxidative stress response (Gara et al., 2003). In plants the main enzymatic antioxidant systems which are activated under stress conditions include:
- SOD: catalyse the dismutation of O2-and HO2 to H2O2. They are metal-containing enzymes (Mn, Fe or Cu/Zn) found in the cytosol, mitochondria, chloroplasts, peroxisomes and glyoxysomes.
- APX: play a role in the scavenging of H2O2.
- ASC: directly scavenges O2- and H2O2 and it is regenerated by dehydroascorbate reductase and at the expense of GSH, which is in turn regenerated by glutathione reductase at the expense of NADPH. GSH can also react directly with OH-. In addition to its role, in ascorbate regeneration,this ascorbate/glutathione cycle is important for the regeneration of á-tocopherol, which is an important antioxidant that scavenges lipid peroxides.
- CAT: is a commonly occurring enzyme converting H2O2 to H2O and O2-. It plays a significant role in reducing H2O2 levels in peroxisomes. Most of the catalases are located in peroxisomes but some may be found in glyoxysomes although a mitochondrial localization is also suggested in some cases.
All the enzymes previously mentioned are present in different isoenzymatic forms in several cell compartments and their expression is genetically controlled, regulated both by developmental, environmental stimuli, according to the necessity to remove ROS produced in cells.
The diversity of enzymatic antioxidant systems present in the cell, constitute their main protection against oxidative damage because of they contribute to a tightly control between ROS production and scavenging. It is believed that the balance between SOD and APX or CAT activities in cells is crucial for determining the steady-state level of O2 and H2O2 (del Río et al., 2002; Gara et al., 2003; Mittler et al., 2004; Shao et al., 2008).
In tobacco, the reduction of catalase and ascorbate peroxidase activities resulted in plants hyper-responsive to pathogens (Mittler et al., 1999), whereas the overexpression of catalase leads to more disease-sensitive plants (Polidoros et al., 2001).
ROS FUNCTION IN PLANT DEFENSE
Plant organisms possess a complex set of defense mechanisms that are responsible for preventing unfavorable interactions with other living organisms in their natural environment or for reducing negative effects of such interactions. Besides, constitutive physical or chemical barriers, plants have developed an array of inducible, local and systemic responses to defend themselves against pathogen attack. They often continually express a range of genes associated with defense against pathogens at low levels, expression of these and additional genes is strongly induced upon contact with specific avirulent races of a pathogen (Shetty et al., 2008).
Subsequently, it was recognised that various plants, including the model plant Arabidopsis , exhibit different oxidative burst phases with an early production of ROS in both compatible and incompatible interactions and a later burst shown only in R -gene-mediated resistance responses (Apel and Hirt, 2004).
In resistant plants, following specific recognition of a pathogen, an early response occur immediately, the hypersensitive response (HR), a plant resistance mechanism leading to cell death, that is notably associated with the generation of ROS in and around the infected cell and afterwards a late response, usually transcription and translation dependent responses that take part in minimizing the long-term effects of the infection and in preventing further infections. These rapid events are transcription independent and they cause morphological and physiological changes in the infected cells and their surroundings. ROS enhancement under stress functions as an alarm signal that triggers acclimatory/defense responses by specific signal transduction pathways that involve H2O2 as secondary messenger (Zaninotto et al., 2006).
Inducible and de-increasing body of data supports the hypothesis that a fine regulation of antioxidant systems is part of the signaling pathways activating defense responses (Laloi et al., 2004). ROS could contribute to the activation of plant defenses by inducing changes in gene expression. The rapidity of its production and the potential for H2O2 to freely diffuse across membranes suggested that, ROS could exert this function either directly through redox regulation of transcription factors or indirectly by interacting with other signaling components like phosphorylation cascades (Mou et al., 2003).
However, the diversity in the systems used for studying plantpathogen interplay makes it difficult to formulate a clear picture of whether and to what extent, changes in antioxidant systems are directly involved in the activation of plant defense responses or are a mere consequence of the oxidative stress occurring in the attacked cells.
For a long time, ROS were considered as dangerous molecules, whose levels are needed to be kept as low as possible. However, it has been realized that these species play important roles in the plants defense system against pathogens (oxidative burst), by their role in tracheary element formation, lignification and other cross-linking processes in the cell wall and act as intermediate signaling molecules to regulate the expression of genes. Because of these multiple functions, it is necessary for cells to control the level of reactive oxygen molecules tightly, but not to eliminate them completely. In particular O2-, its conjugate acid, the perhydroxyl radical (O2H-) and their dismutation product H2O2, which are produced in one or more bursts of oxidative activity during resistance expression in a wide range of host/pathogen interactions due to these species have been seen implicated in stimulation of the HR (Shetty et al., 2008; Van Breusegem et al., 2008).
It is now recognized that various plants, including the model plant Arabidopsis, exhibit different oxidative burst phases with an early production of ROS in both compatible and incompatible interactions and a later burst shown only in R-gene-mediated resistance responses (Apel and Hirt, 2004).
Roles of ROS in hostpathogen interactions
ROS have been implicated in many different processes related to pathogen interactions with their hosts. In the initial phases of the interactions, this essentially means involvement in defence processes, whereas at the later stages, during pathogen colonisation, the role of ROS may be more ambiguous (Shetty et al., 2008).
ROS in biotic interaction
Involvement of ROS in plant responses to pathogen attack has been extensively documented (Torres et al., 2006). Shetty et al. (2003), founded that the infection of wheat by S. tritici was associated with a large and early accumulation of H2O2 in incompatible interactions, coinciding with pathogen arrest and thus indicating a role for H2O2 in the active defense of wheat. Egan et al. (2007) reported that the generation of ROS by the rice blast fungal NADPH oxidase is required for infection. Other authors such us Molina and Kahmann (2007) documented that activation of ROS scavenging mechanisms by the fungal Ustilago maydis Yap1 gene, is essential to overcome the plant defense mechanisms and allow infection. In the same way, Mittapali et al. (2007) reported that the ROS scavenging mechanisms of the Hessian fly (Mayetiola destructor) could play an important function in the interaction with its wheat (Triticum aestivum) host.
A correlation between the apoplastic oxidative burst and the HR has been well documented. Evidences suggest that this is a genetically regulated and active process that has similarities with the animal apoptosis (Lam, 2004). ROS formation normally takes place in the apoplast by the plant NADPH oxidases, but recent reports implicate other sources of ROS in biotic defense responses. These studies highlight apoplastic peroxidases (Bindschedler et al., 2006; Choi et al., 2007) as well as polyamine oxidases (Yoda et al., 2003; Yoda et al., 2006; Angelini et al., 2008) as important for ROS production involved in wound and pathogen responses. Interestingly, ROS
accumulation in response to elicitation was observed in nuclei (Ashtamker et al., 2007), chloroplasts, and mitochondria (Vidal et al., 2007).
In addition, the distribution of iron within the different subcellular compartments was linked to ROS production and activation of plant defenses during the oxidative burst (Liu et al., 2007).
All these studies suggest that ROS production during pathogen responses occurs at multiple subcellular locations (and not exclusively at the apoplast) and that ROS production at these subcellular compartments has an important function in the activation of defense responses and programmed cell death (PCD).
ROS as molecular signaling
There are reasons to believe about the existence of a complex signaling network involving several secondary messengers and providing fine control over the defensive processes on their various levels. It is believed that ROS are an important element of the regulation scheme, together with other secondary messengers like salicylic acid, ethylene, jasmonic acid and nitric oxide (Fujita et al., 2006).
In addition to the necessity of controlling excess potentially damaging ROS, eukaryotes have harnessed ROS as signaling molecules for a diverse array of regulatory processes, including responses to abiotic and biotic stresses, regulation of growth and development, and control of programmed cell death. Because of their important roles as signaling molecules, as well as their toxicity at higher levels, ROS concentrations are finely tuned and developmentally regulated by a complex gene network (at least 289 genes in the model plant Arabidopsis) which collectively control and modulate ROS metabolism (Gadjev et al., 2006; Gechev et al., 2006).
Because of the highly cytotoxic and reactive nature of ROS, their accumulation must be under tight control. That is why higher plants possess very efficient enzymatic and non-enzymatic antioxidant defense systems that allow scavenging of ROS and protection of plant cells from oxidative damage. The distinct subcellular localization and biochemical properties of antioxidant enzymes, their differential inducibility at the enzyme and gene expression level and the plethora of non-enzymatic scavengers render the antioxidant systems a very versatile and flexible unit that can control ROS accumulation temporally and spatially. The above controlled modulation of ROS levels is significant in the light of the recent evidence for a signaling capacity of ROS (Foyer and Noctor, 2005; Gechev et al., 2006; Shao et al., 2008).
Higher plants can sense, transduce and translate the ROS signals into appropriate cellular responses, the process of which requires the presence of redox-sensitive proteins that can undergo reversible oxidation/ reduction and may switch on and off depending upon the cellular redox state. ROS can oxidize the redox-sensitive proteins directly or indirectly via the ubiquitous redox-sensitive molecules, such as GSH or thioredoxins, which control the cellular redox state in higher plants. At the same time, redox-sensitive metabolic enzymes may directly modulate corresponding cellular metabolism, whereas redox-sensitive signaling proteins execute their function via downstream signaling components, such as kinases, phosphatases and transcription factors (Laloi et al., 2004; Mittler et al., 2004; Torres et al., 2006).
In many ways, ROS are ideally suited to be signaling molecules: they are small, and can diffuse short distances; there are several mechanisms for their production, some of which are rapid and controllable and there are numerous mechanisms for their rapid removal. ROS, in particular hydrogen peroxide, are now recognized as important signaling molecules in both the animal and plant kingdoms, being able to cross cellular membranes, is also a diffusible signal for the activation of defense genes and systemic acquired resistance (Kim et al., 2008; Van Breusegem et al., 2008).
Studies have revealed some of the key players in ROS network (Laloi et al., 2004; Mittler et al., 2004) although many questions related to its mode of regulation, its protective roles and its modulation of signaling networks that control growth, development and stress response remain unanswered.
REFERENCES
Able A J, Guest D I, Sutherland M W (2000) Hydrogen peroxide yields during the incompatible interaction of tobacco suspension cells inoculated with Phytophthora nicotianae. Plant Physiology 124: 899-910
Angelini R, Tisi A, Rea G, Chen M M, Botta M, Federico R, Cona A (2008) Involvement of polyamine oxidase in wound healing. Plant Physiology 146 (1): 162-177
Apel K and Hirt H (2004) Reactive oxygen species: metabolism, oxidative stress, and signal transduction. Annual Review of Plant Biology 55: 373-399
Ashtamker C, Kiss V, Sagi M, Davydov O, Fluhr R (2007) Diverse subcellular locations of cryptogein-induced reactive oxygen species production in Tobacco Bright Yellow-2 Cells. Plant Physiology 143 (4): 1817-1826
Asselbergh B, Curvers K, Frana S C, Audenaert K, Vuylsteke M, Van Breusegem F, Höfte M M (2007) Resistance to Botrytis cinerea in sitiens, an abscisic acid-deficient tomato mutant, involves timely production of hydrogen peroxide and cell wall modifications in the epidermis. Plant Physiology 144: 1863-1877
Bechtold U, Karpinski S, Mullineaux P M (2005) The inuence of the light environment and photosynthesis on oxidative signalling responses in plantbiotrophic pathogen interactions. Plant, Cell and Environment 28: 1046-1055
Bindschedler L V, Dewdney J, Blee K A, Stone J M, Asai T, Plotnikov J, Denoux C, Hayes T, Gerrish C, Davies D R, Ausubel F M, Bolwell G P (2006) Peroxidase-dependent apoplastic oxidative burst in arabidopsis required for pathogen resistance. The Plant Journal: 1-13
Breusegem F V, Dat J F (2006) Reactive oxygen species in plant cell death. Plant Physiology 141: 384-390
Choi H W, Kim Y J, Lee S C, Hong J K, Hwang B K (2007) Hydrogen peroxide generation by the pepper extracellular peroxidase CaPO2 activates local and systemic cell death and defense response to bacterial pathogens. Plant Physiology 145: 890-904
Davies D R, Bindschedler L V, Strickland T S, Bolwell G P (2006) Production of reactive oxygen species in Arabidopsis thaliana cell suspension cultures in response to an elicitor from Fusarium oxysporum: implications for basal resistance. Journal of Experimental Botany 57 (8): 1817-1827 del Río L A, Corpas F J, Sandalio L M, Palma J M, Gómez M, Barroso J B (2002) Reactive oxygen species, antioxidant systems and nitric oxide in peroxisomes. Journal of Experimental Botany 53 (372): 1255-1272
Doke N (1983) Involvement of superoxide anion generation in the hypersensitive response of potato tuber tissues to infection with an incompatible race of Phytophthora infestans and to the hyphal wall components Physiologial Plant Pathology 23 (3): 345-357
Egan M J, Wang Z-Y, Jones M A, Smirnoff N, Talbot N J (2007) Generation of reactive oxygen species by fungal NADPH oxidases is required for rice blast disease. PNAS 104 (28): 11772-11777
Foyer C H, Noctor G (2005) Oxidant and antioxidant signalling in plants: a re-evaluation of the concept of oxidative stress in a physiological context. Plant, Cell and Environment 28: 1056 1071
Fujita M, Fujita Y, Noutoshi Y, Takahashi F, Narusaka Y, Yamaguchi-Shinozaki K, Shinozaki K (2006) Crosstalk between abiotic and biotic stress responses: a current view from the points of convergence in the stress signaling networks. Current Opinion in Plant Biology 9 (4): 426-442
Gadjev I, Vanderauwera S, Gechev T S, Laloi C, Minkov I N, Shulaev V, Apel K, Inzé D, Mittler R, Van Breusegem F (2006) Transcriptomic footprints disclose specificity of reactive oxygen species signaling in arabidopsis. Plant Physiology 141: 436-445
Gara L D, Pinto M C d, Tommasi F (2003) The antioxidant systems vis-à-vis reactive oxygen species during plantpathogen interaction. Plant Physiology and Biochemistry 41: 863-870
García-Limones C, Hervás A, Navas-Cortés J A, Jiménez-Díaz R M and Tena M (2002) Induction of an antioxidant enzyme system and other oxidative stress markers associated with compatible and incompatible interactions between chikpea. Physiological and Molecular Plant Pathology 61: 325-337
García-Limones C, Dorado G, Navas-Cortés J A, Jiménez-Díaz R M and Tena M (2009) Changes in the redox status of chickpea roots in response to infection by Fusarium oxysporum f. sp. ciceris: apoplastic antioxidant enzyme activities and expression of oxidative stress-related genes. Plant Biology 11: 194-203
Gechev T S, Van Breusegem F, Stone J M, Denev I and Laloi C (2006) Reactive oxygen species as signals that modulate plant stress responses and programmed cell death. BioEssays 28: 1091-1101 Hancock J T, Desikan R and Neill S J (2001) Role of reactive oxygen species in cell signalling and development. Nature Reviews Molecular Cell Biology 5: 305-315
Lamb C J, Dixon R A (1997) The oxidative burst in plant disease resistance. Annual Review of Plant Physiology and Plant Molecular Biology 48: 251-275
Liu G, Greenshields D L, Sammynaiken R, Hirji R N, Selvaraj G, Wei Y (2007) Targeted alterations in iron homeostasis underlie plant defense responses. Journal of Cell Science 120: 596-605
Mittapalli O, Neal J J, Shukle R H (2007) Tissue and life stage specificity of glutathione s-transferase expression in the Hessian Fly, Mayetiola destructor: Implications for resistance to host allelochemicals. J Insect Sci 7: 20
Mittler R, Vanderauwera S, Gollery M, Van Breusegem F (2004) Reactive oxygen gene network of plants. TRENDS in Plant Science 9 (10): 490-498
Mittler R, Herr E H, Orvar B L, Camp W v, Willekens H, Inzé D, Ellis B E (1999) Transgenic tobacco plants with reduced capability to detoxify reactive oxygen intermediates are hyperresponsive to pathogen infection PNAS 96 (24): 14165-14170
Molina L, Kahmann R (2007) An Ustilago maydis gene involved in H2O2 detoxification is required for virulence. Plant Cell 19 (7): 2293-2309
Mou Z, Fan W and Dong X (2003) Inducers of plant systemic acquired resistance regulate NPR1 function through redox changes. Cell 113: 935-944
Mur L A J, Kenton P, Lloyd A J, Ougham H, Prats E (2007) The hypersensitive response, the centenary is upon us but how much do we know? Journal of Experimental Botany: 1-20
Narusaka Y, Narusaka M, Park P, Kubo Y, Hirayama T, Seki M, Shiraishi T, Ishida J, Nakashima M, Enju A, Sakurai T, Satou M, Kobayashi M,d Shinozaki K (2004) RCH1, a locus in arabidopsis that confers resistance to the hemibiotrophic fungal pathogen Colletotrichum higginsianum. MPMI 17 (7): 749-762
Pignocchi C, Kiddle G, Hernández I, Foster S J, Asensi A, Taybi T, Barnes J, Foyer C H (2006) Ascorbate oxidase-dependent changes in the redox state of the apoplast modulate gene transcript accumulation leading to modified hormone signaling and orchestration of defense processes in tobacco. Plant Physiology 141: 423-435
Polidoros A N, V M P, Scandalios J P (2001) Transgenic tobacco plants expressing the maize Cat2 gene have altered catalase levels that affect plant-pathogen interactions and resistance to oxidative stress. Transgenic Res 10: 555569
Romero D, Rivera M E, Cazorla F M, Codina J C, Fernández-Ortuño D, Torés J A, Pérez-García A, de Vicente A (2008) Comparative histochemical analyses of oxidative burst and cell wall reinforcement in compatible and incompatible melonpowdery mildew (Podosphaera fusca) interaction. Journal of Plant Physiology: 1-11
Ryter S W, Kim H P, Hoetzel A, Park J W, Nakahira K, Wang X, Choi A M K (2007) Mechanisms of cell death in oxidative stress. Antioxidants & Redox Signaling 9 (1): 49-89.
Shao H-B, Chu L-Y, Lu Z-H, Kang C-M (2008) Primary antioxidant free radical scavenging and redox signaling pathways in higher plant cells. Int J Biol Sci 4 (1): 8-14
Shetty N P, Lyngs Jørgensen H J, Due Jensen J, Collinge D B, Shekar Shetty H (2008) Roles of reactive oxygen species in interactions between plants and pathogens. European Journal of Plant Pathology 121: 267-280
Shetty N P, Kristensen B K, Newman M-A, Mollera K, Gregersen P L, Jorgensen H J L (2003) Association of hydrogen peroxide with restriction of Septoria tritici in resistant wheat. Physiological and Molecular Plant Pathology 62 (6): 333-346
Shetty N P, Mehrabi R, Lütken H, Haldrup A, Kema G H J, Collinge D B, Jorgensen H J L (2007) Role of hydrogen peroxide during the interaction between the hemibiotrophic fungal pathogen Septoria tritici and wheat. New Phytologist 174: 637-647
lesak I, Libik M, Karpinska B, Karpinski S, Miszalski Z (2007) The role of hydrogen peroxide in regulation of plant metabolism and cellular signalling in response to environmental stresses. Acta Biochimica Polonica 54 (1): 39-50
Torres M A (2010) ROS in biotic interactions. Physiologia Plantarum 138: 414-429
Torres M A, Jones J D G, Dangl J L (2006) Reactive oxygen species signaling in response to pathogens. Plant Physiology 141: 373-378
Van Breusegem F, Bailey-Serres J, Mittler R (2008) Unraveling the tapestry of networks involving reactive oxygen species in plants. Plant Physiology 147: 978-984
Vidal G, Ribas-Carbo M, Garmier M, Dubertret G, Rasmusson A G, Mathieu C, Foyer C H, Paepe R D (2007) Lack of respiratory chain complex I impairs alternative oxidase engagement and modulates redox signaling during elicitor-induced cell death in T. The Plant Cell Online 19 (2): 640-655
Wientjes F B, Segal A W (1995) NADPH oxidase and the respiratory burst. Seminars in Cell Biology 6 (6): 357-365
Wojtaszek P (1997) Oxidative burst : an early plant response to pathogen infection. Biochem. J. 322: 681-692
Yoda H, Yamaguchi Y, Sano H (2003) Induction of hypersensitive cell death by hydrogen peroxide produced through polyamine degradation in tobacco plants. Plant Physiology 132 (4): 1973-1981
Yoda H, Hiroi Y, Sano H (2006) Polyamine oxidase is one of the key elements for oxidative burst to induce programmed cell death in tobacco cultured cells. Plant Physiology 142 (1): 193-206
Zago E D, Morsa S, Dat J F, Alard P, Ferrarini A, Inzé D, Delledonne M, Van Breusegem F (2006) Nitric oxide- and hydrogen peroxide-responsive gene regulation during cell death induction in tobacco. Plant Physiology 141 (2): 404-411
Zaninotto F, La Camera S, Polverari A, Delledonne M (2006) Cross talk between reactive nitrogen and oxygen species during the hypersensitive disease resistance response. Plant Physiology 141: 379-383
Copyright (c) 2016 Biotecnología Vegetal
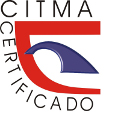
Biotecnología Vegetal eISSN 2074-8647, RNPS: 2154. ISSN 1609-1841, RNPS: 0397 Editada por: Instituto de Biotecnología de las Plantas. Universidad Central Marta Abreu de Las Villas. Carretera a Camajuaní km 5.5, Santa Clara, Villa Clara, Cuba CP 54 830 Tel: 53 42200124, e-mail: info@ibp.co.cu
Biotecnología Vegetal está bajo una Licencia Creative Commons Atribución-NoComercial 4.0 Internacional.