Artículo Científico Biotecnología Vegetal Vol. 10, No. 4: 229 - 236, octubre - diciembre, 2010
ISSN 1609-1841 (Versión impresa)
ISSN 2074-8647 (Versión electrónica)
Expression analysis of three phenylpropanoid-related genes during Musa spp.- Mycosphaerella fijiensis interaction and in response to ethephon
Milady Mendoza-Rodríguez1*, Orelvis Portal1, Bárbara Ocaña1, Mayra Acosta-Suárez1, Berkis Roque1, Monica Höfte2, Elio Jiménez1 *Autor para correspondencia
1Instituto de Biotecnología de las Plantas, Universidad Central Marta Abreu de Las Villas. Carretera a Camajuaní km 5.5, CP 54 830 Santa Clara, Villa Clara. Cuba. e-mail: milady@ibp.co.cu
2 Laboratory of Phytopathology, Faculty of Bioscience Engineering, Ghent University, Coupure Links 653, B-9000 Gent, Belgium
ABSTRACT
Phenylpropanoid pathway is one of the best-studied, most engineered and frequently induced by pathogens or pathogen elicitors. Patterns of expression of chalcone synthase (CHS), flavonoid 35' hydroxylase-like (F35H-like) and isoflavone reductase-like (IFR-like), were analyzed in Calcutta 4 (resistant) and Grande naine (susceptible) plants at 0, 6 and 12 days after artificial inoculation with M. fijiensis and after treatment with 500 ìM ethephon. Reverse transcriptase-polymerase chain reaction (RT- PCR) technique with specific primers was used for this purpose. In the face of fungal challenge F35H-like and IFR-like genes had constitutive expression while CHS gene was induced (in a more significant way in Calcutta 4), in the time range tested for both genotypes. The ethylene producer ethephon induced IFR-like gene in the resistant genotype and in the susceptible one the F35H-like gene. These results will let to continue a deeper study in phenylpropanoid role during Musa-M. fijiensis interaction.
Key words: chalcone synthase (CHS), flavonoid 35' hydroxylase-like (F35H-like), isoflavone reductase-like (IFR-like). Musa spp
RESUMEN
La ruta de los fenilpropanoides es una de las mejor estudiada, más manipulada y frecuentemente inducida por patógenos o elicitores de patógenos. Los perfiles de expresión de chalcona sintasa (CHS), similar a flavonoide 35' hidroxilasa (F35H-like) y similar a la isoflavona reductasa (IFR-like), fueron analizados en plantas de Calcutta 4 (resistente) y Grande naine (susceptible) a los 0, 6 y 12 días posteriores a la inoculación artificial con M. fijiensis y después del tratamiento con etefón 500 ì M. La técnica de reverso transcripción-reacción en cadena de la polimerasa (RT- PCR) con cebadores específicos, fue utilizada para este propósito. En presencia del hongo, los genes F35H-like and IFR-like tuvieron expresión constitutiva mientras que el gen de la chalcona sintasa fue inducido (de una forma mas significativa en Calcutta 4), en el rango de tiempo probado para ambos genotipos. El productor de etileno etefón indujo el gen IFR-like en el genotipo resistente y el gen F35H-like en el susceptible. Estos resultados permitirán continuar profundizando en el estudio del papel de los fenilpropanoides en la interacción Musa-M. fijiensis.
Palabras clave: chalcona sintasa (CHS), Musa spp., similar a flavonoide 35' hidroxilasa (F35H-like), similar a la isoflavona reductasa (IFR-like)
INTRODUCTION
Plants respond to pathogen attack by activating vast arrays of defense reactions: reinforcement of the cell wall (particularly the accumulation of various wall-bound phenolic compounds), changes in ion fluxes, generation of reactive oxygen species (ROS), production of pathogenesis-related proteins and synthesis of a variety of antimicrobial substances (Dixon and Lamb, 1990). A key plant defense mechanisms many pathogens is the synthesis of toxic secondary metabolites, which may be constitutive (phytoanticipins) (VanEtten et al., 1994) or inducible (phytoalexins) (Hammerschmidt, 1999).
Most phytoalexins are derived from the phenylpropanoid metabolism which starts with the oxidation of phenylalanine to cinnamate by phenylalanine ammonia-lyase (PAL) and finishes with the production of a vast array of phenolic derivatives, including the signal molecule salicylic acid (SA). Functional enzymes in this route take part in plant response to wounding and infection directly or indirectly (Arfaoui et al., 2007) and their functions range from preformed or inducible physical and chemical barriers against infection, to signal molecules involved in local and systemic signaling for defense gene induction. Defensive functions are not restricted to a particular class of phenylpropanoid compound, but are found from the simple hydroxycinnamic acids and monolignols to the more complex avonoids, isoavonoids, and stilbenes (Dixon et al., 2002). In higher plants, this pathway generates metabolic intermediates directed to other pathways, including lignin and flavonoids (Treutter, 2006).
Inside phenylpropanoid pathway, the study of the flavonoid branch (including isoflavonoid, a distinctive subclass), has received great attention among scientists. All avonoids are derived from the chalcone scaffold, which is biosynthesized by the ubiquitous plant enzyme CHS, the first committed step of flavonoid biosynthesis in plants. These compounds offer a variety of structural and metabolic functions which induction, in response to stress, has been studied and documented in few model plant systems (mainly legumes) (Treutter, 2005). Their role in plant protection against biotic factors has been seen by many authors (Kim et al., 2003; Saunders and ONeill, 2004; Curir et al., 2005; Zabala et al., 2006; Arfaoui et al., 2007) and in less extension in response to abiotic factors (Winkel-Shirley, 2002; Casati and Walbot, 2003). However, among all these metabolites, isoflavonoids, pterocarpans, isoflavans and isoflavanones of legumes are the best characterized.
In spite of the vast knowledge concerning the role of phenylpropanoid compounds in plant disease resistance (Dixon et al., 2002; Treutter, 2006), their relevance in Musa spp. has not been completely elucidated and it is reduced only to some substances. Horry and Jay (1990), studied flavonol-glycosides (based on quercetin and kaempferol) and anthocyanin-glycosides (based on cyanidin and delphinidin) in Musa species. Collingborn et al. (2000) determined in banana that biochemical basis for nematode resistance is formed by flavan-3,4-diols and condensed tannins. On the other hand, several authors have investigated the activation of phenylpropanoid pathway by measuring PAL activity in: Musa spp.-Mycosphaerella fijiensis pathosystem (Hoss et al., 2000), in Musa spp.-Radopholus similis (Valette et al., 1998) and Musa spp.-Meloidogyne spp. interactions (Wuyts et al., 2006). Finally, some authors have focused on phenylphenalenones and their derivatives in Musaceae, for their potential role as phytoalexins and phytoanticipins (Otálvaro et al., 2007).
The most recent study in Musa spp. on this topic resulted from Portal (2009), who obtained after the construction of a SSH library and a full length cDNA library from Grande naine-M. fijiensis interaction at late stage of infection, the induction of several expressed sequence tags (ESTs) encoding enzymes belonging to phenylpropanoid pathway like CHS, F35H, IFR, chalcone reductase (CHR) and cinnamate-4-hydroxylase (C4H).
Many efforts have been made by scientist trying to understand Musa spp. disease response to Mycosphaerella fijiensis Morelet, which is the causal agent of black leaf streak disease (BLSD), however knowledge related with phenolic compounds as well as with the signal network involved in this biological process is still limited. Taking into account the former results obtained by (Portal, 2009), to continue studying the possible role of secondary metabolites produced throughout phenylpropanoid pathway, during plant-pathogen interaction could be a good start point in the pathosystem understanding. Specifically, to know about expression patterns of some genes involved in flavonoid branch which include isoflavonoid, like CHS, F35H-like and IFR-like, at a transcriptional level, represents the first approximation. At the same time, knowing about the complex network of signaling pathway which mediates banana response to fungal pathogens, after Ethephon elicitation, is another important clue to decipher. The modulation of this network will allow the plant to ne-tune its response to a specific threat and should provide insight into the mechanisms underlying the activation and regulation of defense responses, because of the molecular mechanisms involved in disease response still remain unknown (He et al., 2007).
We approached this problem by monitoring the expression of three genes derived from the phenylpropanoid pathway (CHS, F35H-like and IFR-like) at RNA level by RT-PCR, in banana plants Grande naine and Calcutta 4, after M. fijiensis infection and after ethephon treatment.
MATERIALS and METHODS
Fungal and plant material
The M. fijiensis CCIBP-Pf-83 strain, isolated from a BLSD symptomatic leaf of the susceptible banana genotype Grande naine (Musa AAA) in Santa Clara, Cuba (Cruz et al., 2004), was grown in potato dextrose broth (PDB) medium (Difco), pH 5.6 for 15 days on an orbital rotary shaker (120 rpm) at 28 ºC. The harvested mycelium was prepared by blending in an Ultra Turrax T25 homogenizer (Rose Scientific Ltd.) during one minute and filtered with a 40 μm sieve. The concentration of mycelium fragment suspension was determined by observation under the optic microscope (Leitz Wetzlar) in a Neubauer chamber and it was adjusted approximately to 5.7 x 105 mycelium fragments ml-1. Finally, 1.0% gelatin was added to increase inoculum adhesion to banana leaves.
Two reference cultivars, a highly resistant banana Calcutta 4 (Musa AA) and the susceptible cultivar Grande naine (Musa AAA) (Carlier et al., 2003), were used in this study. Calcutta 4 plants were obtained from the Transit Centre of Bioversity International at Katholieke Universiteit Leuven (Eumusa section with access number ITC0249) and Grande naine plants from in vitro germplasm collection from Instituto de Biotecnología de las Plantas (IBP). Both were propagated in vitro according to the protocol used by INIBAP (Strosse et al., 2004). Finally, rooted plantlets were transferred to plastic pots (one litre capacity) containing 50% casting, 30% compost and 20% zeolite. Afterwards, these were acclimatized in the greenhouse with irrigation three times per day during 45 days, until size reached approximately 20 cm tall and at least four active leaves.
Plant inoculation and sample collection
Banana plants were inoculated with the fungal suspension (four plants from each genotype), according to the protocol described by Alvarado-Capó et al. (2003). After inoculation, high humidity was maintained for three days and the temperature inside the greenhouse was under 30°C with a relative humidity between 60 and 70%. Infected leaves were harvested at 0, 6 and 12 days post inoculation (dpi). Collected samples were immediately frozen in liquid nitrogen and conserved at -80°C for RNA extraction.
Treatment
Ethephon (2-chloroethylphosphonic acid, 500 μM) was sprayed in three banana plants of Calcutta 4 and Grande naine respectively. Control plants were sprayed with distilled water (Laudert and Weiler, 1998). Leave samples were taken at 0, 12, 24 and 48 hours post inoculation. All samples collected were immediately frozen in liquid nitrogen and stored at -80°C, until used for RNA extraction and analysis for expression of F35H-like and IFR-like genes.
RNA extraction and RT-PCR
Total RNA was extracted from leaves following a cetyl trimetyl ammonium bromide (CTAB) based method (Liao et al., 2004). Total RNA after DNAse treatment with TURBO DNAfree Kit (Ambion, UK), was used as templates for RT-PCR that was conducted using the SuperScriptTM III One-step RT-PCR System with Platinum® Taq DNA polymerase (Invitrogen), according to the manufacturers protocol and starting from 150 ng of total RNA.
Primer sets for CHS, F35H-like and IFR-like (Figure 1), were designed with Primer 3 software (Rozen and Skaletsky, 2000), from ESTs of cDNA libraries (including SSH and full-length cDNA library in Grande naine) (Portal, 2009). Primers specific for Musa acuminata actin 1 (based on EF672732.1 GenBank accession number) sequence, were used to check DNA contamination in RNA samples by PCR and as housekeeping gene to normalize the expression levels of the target genes in RT-PCR analysis (Table 1). For each primer pair the optimal annealing temperature was estimated by gradient PCR.
PCR amplifications, using 100 ng of plant genomic DNA as a control and 150 ng of total RNA from infected plant tissue were performed to check the absence of DNA in the RNA samples. The PCR reaction was conducted using 0.5 μM of Ma Actin1 primer pairs, 200 μM dNTPs, 1.5 mM MgCl2 and 1U of Taq DNA polymerase (Fermentas Life Sciences) under the following conditions: Initial denaturalization for 5 min at 94°C, 35 cycles of 30 s at 94°C, 1 min at 59°C and 1 min at 72°C, with a final extensión of 7 min at 72°C. PCR producís were visualized on agarose gel at 1.5% in 1x TBE buffer followed by staining with (EtBr) 0.5 ug.ml1.
RT-PCR conditions were the following: first strand cDNA synthesis at 55 °C 30 min, an initial denaturalization step at 94 °C for 2 min followed by denaturalization for 15s at 94 °C, annealing for 30s at 55 °C and extensión for 30s at 68 °C for 40 cycles with a final extensión of 5 min at 68 °C.
RESULTS
Expression of CHS, F3'5H-like and IFR-like in Calcutta 4 and Grande naine plants after inoculation with M. fijiensis
A constitutive expression of F35H-like and IFR-like, key genes of flavonoid and isoflavonoid pathway respectively, was detected in the time range tested for both genotypes. However, a moderately increased of expression level was detected for IFR-like with respect to F35H-like transcript, after artificial inoculation with M. fijiensis is shown in figure 2A.
There was not CHS expression detected before inoculation. After that there was an increased intensity of CHS expression in the resistant Calcutta 4 genotype compared to the susceptible Grande naine.
F35H-like and IFR-like expression following treatment with ethephon
F35H-like gene was expressed in Calcutta 4 within 48 h of ethephon treatment. In contrast, the induction of this gene occurred earlier in Grande naine since 12 h and transcripts remains high thereafter (Figure 2B).
IFR-like transcript in Calcutta 4 plants reached two peaks of expression at 12 and 48 h. In Grande naine, however, ethephon did not induce gene expression change in response to elicitation.
DISCUSSION
Molecular analysis related with key enzymes of flavonoid (including isoflavonoid) branch, derived from phenylpropanoid pathway, during Sigatoka disease development, constitute an approach to the knowledge of Musa-M. fijiensis pathosystem. It is known that phytoalexins accumulation, mainly those derived from this branch as a result of infection or stress, is the most commonly observed defense reaction in plants (Naoumkina et al., 2007). Their role in plant defense response to fungal and bacterial attack, because of their antimicrobial, antifungal and feeding deterrent properties, have been a continuous subject of study (Treutter, 2006).
The study of active phytoalexins produced via phenylpropanoid pathway in defense to phytopathogenic organisms in Musa genus, has received less attention and it is reduced only to phenylphenalenones (Otálvaro et al., 2002; Otálvaro et al., 2007). In spite of the current knowledge about the activation of this pathway, very little has been concluded regarding gene activities of enzymes of the flavonoid and isoflavonoid branches, leading to the synthesis of different phytoalexins in response to M. fijiensis infection.
The present study shows that inoculation with M. fijiensis favor CHS gene transcript accumulation, during compatible as in the incompatible interaction and after plant elicitation F35H-like or IFR-like induction, was close related to the resistant phenotype, which is described for the first time.
Based on the differences in transcripts levels, some authors have showed that it could be correlated with the differences in the susceptibility/resistance of the host plant to fungal disease (Wang et al., 2006). Others have found that in both resistance phenotypes a defense response is achieved but the main differences are established between time of recognition of pathogen attack and the rapid appropriate expression of defense responses (Umemura et al., 2003).
The study of differential expression patterns from genes derived from phenylpropanoid pathway, has provided an opportunity to understand the biochemical and molecular basis involved in plant-pathogen interaction. Several authors have studied the role of one or several genes encoding key enzymes of this pathway, during plant response to biotic stress and have found some evidence to understand host-pathogen interaction, throughout the elucidation of the biochemical pathways that are needed for both, pathogenesis and resistance in pathogen and plants, respectively.
There are several examples documenting direct transcription rates of phenylpropanoid pathway-derived genes, following plant infection and after applying elicitors.
Saunders and ONeill (2004), observed that the defensive plant protection response in Medicago sativa to Colletotrichum trifolii, was accompanied by an increase in PAL, cinnamic acid 4-hydroxylase (CA4H) and IFR mRNA transcripts, followed by the accumulation of medicarpin and sativan. Chickpea seedlings inoculated with Fusarium oxysporum, Arfaoui et al. (2007) showed that the increase accumulation of phenolics compounds were in correspondence with the increase expression of three genes controlling key phenylpropanoid enzymes PAL, CHS and IFR.
Regarding IFR/IFR-like gene, which has been taken into account in a vast majority of scientific research, a grapefruit IFR-like was induced by the fungal pathogen Penicillium digitatum and by wounding, but more slowly and to a lesser extent by UV irradiation (Lers et al., 1998). However Kim et al. (2003) obtained the induction of OsIFR-like by fungal elicitor, jasmonic acid (JA) and also in compatible and incompatible interactions when pathogen was co-cultured with rice suspension cells. Specifically in Musa genus, Kesari et al. (2007) and Portal (2009) found an isoflavone reductase, among all genes expressed differentially throughout the PCR-based suppression subtractive hybridization (SSH) technique, in ripe banana and after Grande naine infected plants at a late stage of infection with M. fijiensis, respectively.
Another important gene studied is CHS. In Phaseolus vulgaris-Colletotrichum lindemuthianum pathosystem, it was found CHS induction, which indicated that control of phytoalexin gene expression is a key early component in the defense response (Bell et al., 1984). Zabala et al. (2006) during the study of Glycine max response to Pseudomonas syringae, found that CHS transcript were strongly upregulated in response to pathogen attack and the induction of isoflavonoid branch of phenylpropanoid pathway.
All these results confirmed the significance of the study phenylpropanoids derived genes to unravel specific plant-pathogen interaction and discover new candidates of genes related with resistance to fungal diseases.
The interaction between plant pathogens and their hosts involves a continuous molecular dialogue between the two organisms. Disease resistance is regulated by multiple signal transduction pathways like ethylene (ET), (JA), (SA), and abscisic acid (ABA), which function as key signaling molecules. Deciphering this «crosstalk» between these hormones-dependent pathways in plant cells is a major challenge taking in to account the possibility to understand how the cell orchestrates an optimal response to a specific biotic stress.
The current aspects has been used in previous scientific researches and in the case of ethylene, with respect to pathogen defense, it is involved in the early responses whereas JA and SA may control prolonged effects (De Paepe et al., 2004) .
More studies are necessary to dissect and understand the complex mechanisms involved in gene regulation during banana defense response to pathogens such as M. fijiensis. Further chemical and biological studies might elucidate the possible role of avonoids in disease resistance in Musa. Specifically to understand how IFR-like gene is activated, insights into the mechanisms underlying the coordinated transcriptional activation of a complex secondary biosynthetic pathway and the evolution of the isoflavonoid branch of flavonoid metabolism, as a defense mechanism in Musa spp., versus M. fijiensis, will be provided although very little is known about signal transduction pathways, transcriptional regulation and molecular mechanisms underlying the induction of the isoflavonoid branch pathway.
CONCLUSIONS
The present study showed that inoculation with M. fijiensis can result in gene transcript accumulation of CHS during compatible as in the incompatible interaction. Besides, induction of IFR-like in the resistant genotype and in the susceptible one the F35H-like gene, which is described for the first time was observed after plant elicitation.
REFERENCES
Alvarado-Capó Y, Leiva M, Dita Rodríguez MA, Acosta M, Cruz M, Portal N, Gómez R, García LR, Bermúdez I, Padrón Y (2003) Early evaluation of black leaf streak resistance by using mycelial suspensions of Mycosphaerella fijiensis. In: Jacome L, Lepoivre P, Marín DH, Ortiz R, Romero RA and Escalant J-V (Eds.) Mycosphaerella leaf spot diseases of bananas: present status and outlook. Proceedings of the 2nd International Workshop on Mycosphaerella leaf spot diseases. INIBAP, San José, Costa Rica. pp. 169-175
Arfaoui A, El Hadrami A, Mabrouk Y, Si B, Boudabous A, El Hadrami I, Daayf F, Chérif M (2007) Treatment of chickpea with Rhizobium isolates enhances the expression of phenylpropanoid defense-related genes in response to infection by Fusarium oxysporum f. sp. ciceris. Plant Physiology and Biochemistry 45: 470-479
Bell JN, Dixon RA, Bailey JA, Rowel PM, Lamb CJ (1984) Differential induction of chalcone synthase mRNA activity at the onset of phytoalexin accumulation in compatible and incompatible plant-pathogen interactions. Proc. Natl. Acad. Sci. USA 81: 3384-3388
Carlier J, De Waele D, Escalant J-V (2003) Global evaluation of Musa germplasm for resistance to Fusarium wilt, Mycosphaerella leaf spot diseases and nematodes. In: Vézina A and Picq C (Eds.) INIBAP Technical Guidelines 7. The International Network for the Improvement of Banana and Plantain. Montpellier, France
Casati P, Walbot V (2003) Gene expression profiling in response to ultraviolet radiation in maize genotypes with varying flavonoid content. Plant Physiology 132 (4): 1739-1754
Collingborn FMB, Gowen SR, Mueller-Harvey I (2000) Investigations into the biochemical basis for nematode resistance in roots of three Musa cultivars in response to Radopholus similis infection. J. Agric. Food Chem 48 (11): 5297-5301
Cruz-Martín M, Alvarado-Capó Y, Acosta-Suárez M, Leiva-Mora M, Roque B (2004) Caracterización de aislados de Pseudocercospora fijiensis Morelet para su utilización en programas de mejoramiento de Musa spp. Biotecnología Vegetal 4 (2): 111-114
Curir P, Dolci M, Galeotti F (2005) A phytoalexin-like flavonol involved in the carnation (Dianthus caryophyllus)-Fusarium oxysporum f. sp. dianthi pathosystem. J. Phytopathology 153: 65-67
De Paepe A, Vuylsteke M, Van Hummelen P, Zabeau M, Van Der Straeten D (2004) Transcriptional proling by cDNA-AFLP and microarray analysis reveals novel insights into the early response to ethylene in arabidopsis. The Plant Journal 39: 537-559
Dixon RA, Lamb CJ (1990) Molecular communication in interactions between plants and microbial pathogens. Annu. Rev. Plant. Physiol. Plant Mol. Biol. 41: 339-367
Dixon RA, Achnine L, Kota P, Liu C-J, Srinivasa Reddy MS, Wang L (2002) The phenylpropanoid pathway and plant defence-a genomics perspective. Molecular Plant Pathology 3 (5): 371-390
Hammerschmidt R (1999) Phytoalexins: What have we learned after 60 years. Ann. Rev. Phytopathol. 37: 285-306
He P, Shan L, Sheen J (2007) Elicitation and suppression of microbe-associated molecular pattern-triggered immunity in plantmicrobe interactions. Cellular Microbiology 9 (6): 1385-1396
Horry J-P, Jay M (1990) An evolutionary background of bananas as deduced from flavonoids diversification. En: Jarret RL (Ed.) Identification of genetic diversity in the genus Musa. Proceedings of an international workshop. Los Baños, Philippines, 5-10 september 1988. pp. 41-55
Hoss R, Helbig J, Bochow H (2000) Function of host and fungal metabolites in resistance response of banana and plantain in the black Sigatoka disease pathosystems (Musa spp.-Mycosphaerella fijiensis). Journal of Phytopathology 148: 387-394
Kesari R, Kumar Trivedi P, Nath P (2007) Ethylene-induced ripening in banana evokes expression of defense and stress related genes in fruit tissue. Postharvest Biology and Technology 46: 136-143
Kim ST, Cho KS, Kim SG, Kang SY, Kang KY (2003) A rice isoflavone reductase-like gene, OsIRL, is induced by rice blast fungal elicitor. Mol. Cells 16 (2): 224-231
Laudert D, Weiler EW (1998) Allene oxide synthase: A major control point in Arabidopsis thaliana octadecanoid signaling. Plant J. 15: 675-684
Lers A, Burd S, Lomaniec E, Droby S, Chalutz E (1998) The expression of a grapefruit gene encoding an isoavone reductase-like protein is induced in response to UV irradiation. Plant Molecular Biology 36: 847-856
Liao Z, Chen M, Gong Y, Tang F, Sun X, Tang K (2004) Rapid isolation of high quality total RNA from Taxus and Ginkgo. Preparative Biochemistry and Biotechnology 34 (3): 209-214
Naoumkina MA, Farag MA, Sumner LW, Tang Y, Liu C-J, Dixon RA (2007) Different mechanisms for phytoalexin induction by pathogen and wound signals in Medicago truncatula. PNAS 104 (46): 17909-17915
Otálvaro F, Echeverri F, Quiñones W, Torres F, Schneider B (2002) Correlation between phenylphenalenone phytoalexins and phytopathological properties in Musa and the role of a dihydrophenylphenalene triol. Molecules 7: 331-340
Otálvaro F, Nanclares J, Vásquez LE, Quiñones W, Echeverri F, Arango R, Schneider B (2007) Phenalenone-type compounds from Musa acuminata var. Yangambi km5 (AAA) and their activity against Mycosphaerella fijiensis. Journal of Natural Products 70: 887-890
Portal O (2009) Development of molecular tools to study the interaction between banana and Mycosphaerella fijiensis, the causal agent of black leaf streak disease. PhD Thesis. Department of Crop Protection, Laboratory of Phytopathology. Universiteit Gent, Gent. 162 p.
Rozen S, Skaletsky H (2000) Primer 3 on the WWW for general users and for biologist programmers. Humana Press Inc. Totowa, NJ
Saunders JA, ONeill NR (2004) The characterization of defense responses to fungal infection in alfalfa. BioControl 49: 715-728
Strosse H, Van den Houwe I, Panis B (2004) Banana cell and tissue culture-review. Science Publishers. Enfield (NH), USA and Plymouth, UK
Treutter D (2005) Significance of flavonoids in plant resistance and enhancement of their biosynthesis. Plant Biology 7: 581-591
Treutter D (2006) Signicance of avonoids in plant resistance: a review. Environ. Chem. Lett. 4: 147-157
Umemura K, Ogawa N, Shimura M, Koga J, Usami H, Kono T (2003) Possible role of phytocassane, rice phytoalexin, in disease resistance of rice against the blast fungus Magnaporthe grisea. Biotechnology and Biochemistry 67: 899-902
Valette C, Andary C, Geiger JP, Sarah J-L, Nicole M (1998) Histochemical and cytochemical investigations of phenols in roots of banana infected by the burrowing nematode Radopholus similis. Phytopathology 88 (11): 1141-1148
VanEtten HD, Manseld JW, Bailey JA, Farmer EE (1994) Two classes of plant antibiotics: phytoalexins versus phytoanticipins. The Plant Cell 6: 1191-1192
Wang X, El Hadrami A, Adam LR, Daayf F (2006) Local and distal gene expression of pr-1 and pr-5 in potato leaves inoculated with isolates from the old (US-1) and the new (US-8) genotypes of Phytophthora infestans (Mont.) de Bary. Environmental and Experimental Botany 57: 70-79
Winkel-Shirley B (2002) Biosynthesis of flavonoids and effects of stress. Current Opinion in Plant Biology 5: 218-223
Wuyts N, De Waele D, Swennen R (2006) Activity of phenylalanine ammonia-lyase, peroxidase and polyphenol oxidase in roots of banana (Musa acuminata AAA, cvs Grande Naine and Yangambi km5) before and after infection with Radopholus similis. Nematology 8 (2): 201-209
Zabala G, Zou J, Tuteja J, Gonzalez DO, Clough SJ, Vodkin LO (2006) Transcriptome changes in the phenylpropanoid pathway of Glycine max in response to Pseudomonas syringae infection. BMC Plant Biology 6: 1-45
Copyright (c) 2016 Biotecnología Vegetal
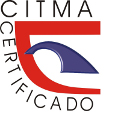
Biotecnología Vegetal eISSN 2074-8647, RNPS: 2154. ISSN 1609-1841, RNPS: 0397 Editada por: Instituto de Biotecnología de las Plantas. Universidad Central Marta Abreu de Las Villas. Carretera a Camajuaní km 5.5, Santa Clara, Villa Clara, Cuba CP 54 830 Tel: 53 42200124, e-mail: info@ibp.co.cu
Biotecnología Vegetal está bajo una Licencia Creative Commons Atribución-NoComercial 4.0 Internacional.