Artículo original
Biotecnología Vegetal Vol. 16, No. 2: 91 - 101, abril - junio, 2016
Instituto de Biotecnología de las Plantas. UCLV. MES.
eISSN 2074-8647, RNPS: 2154
Soil microbial community response to different farm managements in Santa Clara, Cuba
Respuesta de la comunidad microbiana del suelo a manejos agrícolas diferentes en Santa Clara, Cuba
Yanetsy Ruiz-González1, Edith Aguila-Alcantara1, Osvaldo Fernández-Martínez2, David Buchan3, Bram Moeskops3, Marijke D’Haese4, Stefaan De Neve3
1Departamento de Agronomía, Facultad de Ciencias Agropecuarias, Universidad Central Marta Abreu de Las Villas. Carretera a Camajuaní km 5,5. Santa Clara. Villa Clara. Cuba. CP 54 830.
e-mail: editha@uclv.edu.cu
2Instituto de Biotecnología de las Plantas, Universidad Central Marta Abreu de Las Villas. Carretera a Camajuaní km 5,5. Santa Clara. Villa Clara. Cuba. CP 54 830.
3Department of Soil Management, Ghent University. Coupure Links 653. Gent. Belgium. 9000.
4Department of Agricultural Economics, Ghent University. Coupure Links 653. Gent. Belgium. 9000.
ABSTRACT
Seven farms (two state, two cooperatives, and three private farms) were selected for assessing effects of farm management on the microbial biomass and the structure of the microbial community, as well as, the responses to seasonality on these two bio-indicators in these three representative farming systems. All farms are located on brown calcareous soil. Soil samples from the 0-20 cm depth were collected from two fields of each farm. Soil microbial community was assessed through two analyses: microbial biomass carbon and phospholipid fatty acid. The technological differences in soil management, among the three farming systems, affected both microbial biomass carbon and the microbial community composition. The differences were most pronounced between the private and the state farms. The statistical analyses demonstrated that the total of phospholipid fatty-acid were significantly higher in cooperative farms. The use of fallow in these farms seems to have positive effects on soil microbial communities. Seasonality has a clear effect on both indicators. Summarizing, both indicators demonstrated sensible responses to disturbances caused by farm management and seasonality in the conditions of Cuban agriculture.
Keywords: farming systems, microbial biomass carbon, phospholipid fatty-acid analysis (PLFA), soil quality
RESUMEN
Siete fincas (dos estatales, dos cooperativas y tres privadas) fueron seleccionadas para evaluar los efectos del manejo agrícola en la biomasa microbiana y en la estructura de la comunidad microbiana, así como, la respuesta a la estacionalidad de estos dos bio-indicadores en los tres sistemas agrícolas. Todas las fincas se encontraban sobre suelos pardos con carbonatos. Las muestras fueron colectadas en dos campos de cada finca a una profundidad de 0-20 cm. La comunidad microbiana fue evaluada a través de dos análisis: carbono de la biomasa microbiana y los fosfolípidos. Las diferencias tecnológicas en el manejo de los suelos, entre los tres sistemas agrícolas, afectaron el carbono de la biomasa microbiana y la estructura de la comunidad microbiana. Las diferencias fueron más pronunciadas entre los sistemas privados y estatales. Los análisis estadísticos demostraron que el total de los fosfolípidos fue significativamente superior en los campos de las cooperativas. El uso del barbecho en estas fincas parece tener efectos positivos en la comunidad microbiana del suelo. La estacionalidad tuvo un claro efecto en los dos indicadores. En resumen, estos bioindicadores demostraron respuestas sensibles a los disturbios causados por el manejo y la estacionalidad en las condiciones de la agricultura cubana.
Palabras clave: análisis de ácidos grasos fosfolípidos (PLFA), calidad del suelo, carbono de la biomasa microbiana, sistemas agrícolas
INTRODUCTION
Soils are complexes, diverse, and heterogeneous and provide several important functions to the ecosystems (García-Orenes et al., 2013). Nowadays, improving of soil quality constitutes the base for enhancing food security and ecosystem functioning. From this perspective soil, biology has been addressed as a crucial indicator of soil quality, due to the major role of soil biota in organic matter turnover and because of the quick response of biological soil properties to changes in soil caused by crop management practices (D´Hose et al., 2014).
Microbial habitat is intimately related to soil functioning and its genesis (Gupta et al., 2008). Microorganisms play an important role in nutrient cycling, mineralization of nutrients, soil aggregation; hence, they are important for the maintenance of soil fertility and the sustainability of the ecosystems and any kind of agriculture (Esperschütz et al., 2007).
The composition of SMC may be influenced by environmental conditions, vegetation and soil properties (Hackl et al., 2005), but also cultivation (McKinley and White, 2005; Moore-Kucera and Dick, 2008).
The soil microbial biomass (SMB) represents a significant compartment of the terrestrial biomass and microbial residues in soil are an important source material for humus formation (John, 2003). As active fraction, SMB changes continually and responds much more rapidly to changes in the environment than total organic matter, being reported as an important sensitive indicator to environmental stress and changes in agricultural managements (Campos, 2008).
Moreover, agricultural management influences soil microbial communities, altering their functioning and structure, which in turn may have significant implications for soil quality. Management practices have a direct effect on soil microbiota. For assessing changes in soil microbiota due to agricultural has been used the phospholipid fatty acid (PLFA) analysis. The PLFA method is very useful and efficient when assessing soil microbiota composition; using the lipids of the microbial membranes as biomarkers of specific groups of microorganisms; which permit to analyze the profile of the microbial community structure (Frostegard et al., 2011; García-Orenes et al., 2013).
In Cuba, farming systems can be grouped into three major groups: private, cooperative, and state farms. These three groups differ in land ownership, technological complexity and agricultural intensification. State farms comprise large areas and follow a conventional agriculture model, producing food for the satisfaction of social demands (schools, hospitals, and also, population). These farms have access to improved seeds, fuel, chemical fertilizers, pesticides and mechanized technologies for soil preparation and harvesting (McCune et al., 2011).
The cooperative farms are formed by the aggregation of several private farmers. In these farms, farming is done under similar organizational structures as the large state farms. Their aim is also producing food for distribution on a regional scale. The average size of cooperative farms range from 350 to 550 hectares (ONE, 2010). Private farms are owned and managed by independent farmers producing food for local consumption. These farms are characterized by a small production scale, high crop diversity, the use of alternative technologies for agricultural production, such as animal traction and intensive crop rotation, and the applications of intercropping (Figueroa, 2002; Cruz, 2005).
The impacts of farm management on soil microorganisms and carbon storage potential has been poorly explored (Moore-Kucera and Dick, 2008). In Cuba, studies approaching the influence of farm management on soil biological indicators are scarce in the country. The goal of this research was to assess the effects of different farm managements on SMB and SMC composition, as well as, the responses to seasonality on these two bio-indicators in these three representative farming systems.
MATERIALS AND METHODS
Description of the area and experimental design
This study was carried out in Santa Clara municipality, Villa Clara province, Cuba. Santa Clara has a tropical climate with a humid summer (Aw). The rainy season starts in May and ends in October. Annual rainfall is 1100 mm on average, and the 80% of the total precipitation falls in the rainy season. Mean temperature is about 24 degrees, the hottest months are June, July and August, and the coolest is January. Mean relative humidity is usually around 81% and can go up to 86% (CITMA, 2008).
All selected farms were located on brown calcareous soil classified as orthi-calcaric cambisol in the FAO-UNESCO system (Hernández, et al., 2005). The cambisols are the most common soil group throughout the country, accounting for 2 355 800 ha that represent 26.99% of all agricultural land in Cuba. Particularly in Villa Clara, this soil type occupies 249 400 ha, representing 33.29% of all productive lands (INS, 1999).
In Santa Clara, there are more private farms than state and cooperative farms. In the soil type selected for the study, private farms are more distributed throughout the municipality; meanwhile the state and cooperative farms are located in the northwest and northeast of Santa Clara city. Due to the uneven distribution of the private farms, three of them were selected for the research, but only two cooperative and two state farms (seven in total).The main characteristics of the technologies used by farming systems are described in table 1.
The main crops, crop rotations and field cover in the moment of the study are described by fields in table 2.
Soil sampling
Soil sampling was done in two consecutive years (2009 and 2010), during the rainy season (September) and the dry season (April). At each farm, two representative fields of 1-2 ha were selected for the research. Three composites samples were collected by field. Each sample was formed by mixing and homogenizing soil from 6 cores, of 20 cm depth each. The analyses were done in laboratories of the Department of Soil Management at the University of Ghent in Belgium. Soil sampling was done 24 h before transporting the samples to Belgium. The samples were kept in the freezer until laboratory analyses.
Soil biological analysis
Microbial Biomass Carbon (MBC)
The MBC was determined using the fumigation-extraction technique (Jenkinson and Powlson, 1976; Vance et al., 1987; Joergensen, 1996). Both fumigated soil and un-fumigated controls (25 g) were extracted in duplicate with 50 ml 0.5 M K2SO4. Extracts were stored at -18°C until analysis. Organic carbon (OC) contents of the extracts were determined with a Total Organic Carbon (TOC) analyzer (TOC-VCPN, Shimadzu Corp., Kyoto, Japan). For conversion from OC contents in the extracts to MBC in the soil a kEC value of 0.35 was assumed (Joergensen, 1996).
Phospholipids fatty-acid (PLFA) analysis
The structure of the microbial community was described by the fatty acid composition of the phospholipids in the soil. Determination of PLFA followed a procedure modified from Balser (2001). The procedure requires avoid light, oxygen, water and heat, which will destroy fatty acids. Four gram freeze-dried soil was weighed in glass centrifuge tubes. Then, 3.6 ml phosphate buffer pH 7.0, 4 ml chloroform and 8 ml methanol were added. The tubes were shaken for 1 h and afterwards centrifuged for 10 min at a centrifugal force of 699 x g. The supernatant was decanted in new glass tubes and 3.6 ml phosphate buffer and 4 ml chloroform were added. Samples were left overnight for phase separation, cover with aluminum foil and away from heat sources. The next day, the lipid layer was transferred to smaller glass tubes. The remaining phase was washed with 3 ml chloroform to remove any remaining lipids. The combined lipid fraction was dried under N2. Phospholipids were separated from the lipid extracts by solid phase extraction, using silica columns (Chromabond, Macherey-Nagel GmbH, Düren, Germany). After discarding neutral and glycolipids by chloroform and acetone respectively, phospholipids were eluted using methanol. The methanol fraction was dried under N2. The dried phospholipids were then dissolved in 1 ml methanol:toluene (1:1 vol:vol) and 1 ml 0.2 M methanolic KOH. Samples were incubated at 35°C for 15 min to allow transesterification to methyl esters. After cooling to room temperature, 2 ml hexane:chloroform (4:1 vol:vol), 1 ml 1 M acetic acid and 2 ml water were added to the tubes. After vortexing, the samples were centrifuged for 5 min at a centrifugal force 447 x g. The hexane layer, containing the methylated PLFAs, was transferred to pointed glass tubes. The aqueous phase was washed twice with hexane:chloroform for removing any remaining lipids. The combined hexane phase was dried under N2. The fatty-acid methyl esters were finally dissolved in 0.3 ml hexane containing methyl nonadecanoate fatty acid (C19:0) as an internal standard. PLFAs were determined by GC-MS on a Thermo Focus GC combined with a Thermo DSQ quadrupole MS (Interscience BVBA, Louvain-la-Neuve, Belgium) in electron ionization mode. Samples were injected on a Varian capillary column CP Sil 88 (100 m x 0.25 mm i.d., 0.2 μm film thickness; Varian Inc., Palo Alto, USA). The PLFA markers used for calculating the microbial groups are listed in table 3.
Data analysis
For the MBC, results were tested for each season independently using one way ANOVA analysis by Tukey test (P<0.05).
The relative composition of the microbial community was calculated as average value of the total PLFA concentration by microbial group in each farming system. The final value used was expressed in nmol g-1 of dry soil. Significant differences among the total amounts of PLFA per farming system for each season were tested using the Bonferroni test at significance level of P<0.05.The variability was expressed by the Principal Components Analysis (PCA) using biplots and vector graphs. This analysis was performed for each season. All the statistical analyses were performed using SPSS v17 and Statgraphics Centurion, as well as Microsoft Excel 2007.
RESULTS AND DISCUSSION
Microbial Biomass Carbon
Fields on private farms had significantly higher MBC in both seasons than state farms in all field, with the exception of only one field. The MBC tended to be higher also in private farms than in cooperative farms, but this was only significant in 50% of the cases (Figure 1). This result suggests a significant effect of the farm management on this soil quality indicator.
The results demonstrated the farm management has significant influences on MBC and on soil microbial communities in general. Similar results have been reported in the literature (Balota and Martins, 2011; Oyedele et al., 2015; Amaral and Abelho, 2016). Several authors have reported the negative effects of agricultural practices on MBC (Enkenler and Tatabai, 2003; Alvear et al., 2006). In this case, the differences among these three farming systems related to soil management seems to have direct effects on SMB. In the state farms, the intensive cropping, high tillage intensity, application of agricultural chemicals and limited crop rotation among other agricultural practices affect soil organic matter quality and; therefore, soil MBC.
A higher MBC in private farms may indicate greater carbon accumulations in the organic pool, and could represent either a sink or a source of plant-available nutrients, depending on the soil management. Different practices such as intercropping, different cover species and tillage systems have been pointed out due to their significant effects on MBC (Balota and Martins, 2011; Oyedele et al., 2015).
Long-term organic farming, but also, low input farming have been pointed out to be beneficial to MBC and soil biota in general. The regular application of organic fertilizers provides easily available carbon sources, which favor an increment in soil microbial communities (Esperschütz et al., 2007).
PLFAs by farming systems and seasons
Differences in the total amount of PLFA between farming systems were strongly dependent on the season. In the rainy season, there were no differences at all between the farming systems (only a much larger variability in the private farming fields), whereas in the dry season, the three farming systems were significantly different, with the largest total PLFA in the cooperative farming fields (Figure 2). In that sense, the total PLFA concentration did not follow the same trend as MBC, where often private farming fields had the highest values of these indicators.
The PCA analysis based on the PLFAs (Figures 3,4) also showed a very different image between the rainy and the dry season. During the dry season, there was very little discrimination between the PLFA profiles for the different farming systems. The state and cooperative fields are concentrated in a small area of the PCA, and partially overlap, but are completely enveloped by the private fields, which showed a much larger spread. In the rainy season, the farming systems are almost completely separated and are spread mainly along the PC1 axis. Once again, the private farming fields show the highest spread, but much less than in the dry season. The factor loadings showed that no particular fatty acid had a much more pronounced influence than other fatty acids. All contributed equal to the PC’s, and pointing all in the same direction along the PC1 axis.
The different trends found of PLFA and MBC is particularly surprising, since normally a good correlation is expected between MBC and PLFA (both are a measure of microbial biomass) (Bailey et al., 2002; Rinklebe and Langer, 2010; Kujur and Patel, 2014).
It was not found a direct explanation for these discrepancies in soil biological parameters. In the cooperative farms, there is a practice of fallow periods. It has been shown that discontinuation of cultivation can be a factor with positive influence on soil quality (McKinley and White, 2005). However, then it would be found an effect on MBC as well, and not on PLFA only. The fact that differences in total PLFA were only visible in the dry season is not in contradiction with the strongest differences in dry season also in MBC. However, this is also surprising because in the dry season very little microbial activity would be expected at all.
PLFA profiles have been used previously with success to discriminate between farming systems. Moeskops et al. (2010) found a very clear discrimination between intensive conventional and organic vegetable farming systems in West Java, Indonesia. In this study, no such strong discriminating power of PLFAs was observed. This may be because these farming systems probably differ less in management and inputs than those very intensive conventional and organic vegetable production systems from the study of Moeskops et al. (2010).
CONCLUSIONS
The technological differences in soil management, among the three farming systems, affected both SMB and SMC composition. The differences in the composition of soil microbial communities were most pronounced between the private and the state farms. The statistical analyses demonstrated that the concentrations of marker fatty acid, that describe the total PLFA of this study, were significantly higher in cooperative farms. The use of fallow in these farms seems to have positive effects on soil microbial communities. Seasonality has a clear effect on microbial communities (MBC, PLFA). The high sensitivity of these biomarkers under Cuban climatic conditions suggests that they can be a useful tool for assessing soil microorganism responses to farm management.
ACKNOWLEDGEMENTS
This research was supported by the project 5 “Improving the quality of graduate and post-graduate education and research programs in plant and animal sciences” of the IUC program VLIR - UCLV.
REFERENCES
Amaral F, Abelho M (2016) Effects of agricultural practices on soil and microbial biomass carbon, nitrogen and phosphorus content: a preliminary case study. Web Ecol 16: 3–5; doi: 10.5194/we-16-3-2016
Alvear M, Mario B, Carlos R, Trasar-Cepeda C, Gil-Sotres F (2006) Efecto de la cero labranza sobre algunas actividades biológicas en un alfisol del sur de Chile. J Soil Sc Plant Nutr 6 (2): 38 – 53; doi: 10.4067/S0718-27912006000200004
Bailey VL, Peacock AD, Smith JL, Bolton Jr H (2002) Relationships between soil microbial biomass determined by chloroform fumigation – extraction, substrate-induced respiration, and phospholipid fatty acid analysis. Soil Biology & Biochemistry 34: 1385–1389; doi: 10.1016/S0038-0717(02)00070-6
Balota E L, Martins A (2011) Soil microbial biomass under different management and tillage systems of permanent intercropped cover species in an orange orchard. R Bras Ci Solo 35:1873-1883; doi: 10.1590/S0100-06832011000600004
Balser TC (2001) The impact of long-term nitrogen addition on microbial community composition in three Hawaiian forest soils. TheScientificWorld 1 (S1): 500-504; doi: 10.1100/tsw.2001.450
Campos A (2008) Biological and biochemical transformation of nutrients in agricultural soils of Yucatan, Mexico. PhD Thesis. University of Goettingen, Gottingen, Germany
CITMA (2008) Manual de Procedimiento de Información Meteorológica. Instituto Meteorológico, Villa Clara
Cruz H (2005) ¿De qué suelos esperamos alimentarnos? Temas 44: 35-47
D’Hose T, Molendijk L, van den Berg W, Hoek H, Runia W (2014) Impacts of soil management on biological soil quality. Final report CATCH-C project (Grant Agreement N° 289782). Disponible en: http://cordis.europa.eu/docs/results/289/289782/final1-pubsum-final.pdf Consultado 15/12/2015
Enkenler M, Tatabai M A (2003) Effects of liming and tillage systems on microbial biomass and glycosidases in soils. Biol Fertil Soils 39 (1):51–61; doi: 10.1007/s00374-003-0664-8
Esperschütz J, Gattinger A, Mäder P, Schloter M, Fließbach A (2007) Response of soil microbial biomass and community structures to conventional and organic farming systems under identical crop rotations. FEMS Microbiology Ecology 61 (1): 26-37; doi: 10.1111/j.1574-6941.2007.00318.x
Figueroa V (2002) Reforma estructural del régimen agrario de la transición socialista en Cuba. GEDERCO UCLV, Santa Clara
Frostegard A, Tunlid A, Baath E (2011) Use and misuse of PLFA measurements in soils. Soil Biology & Biochemistry 43 (8): 1621-1625; doi: 10.1016/j.soilbio.2010.11.021
García-Orenes F, Morugán-Coronado A, Zornoza R, Scow K (2013) Changes in soil microbial community structure influenced by agricultural management practices in a mediterranean agro-ecosystem. PLoS ONE 8 (11): e80522; doi: 10.1371/journal.pone.0080522
Gupta VVSR, Dick RP, Coleman DC (2008) Functional microbial ecology: molecular approaches to microbial ecology and microbial habitats. Soil Biology & Biochemistry 40 (6): 1269–1271; doi: 10.1016/S0038-0717(08)00044-8
Hackl E, Pfeffer M, Donat C, Bachmann G, Zechmeister-Boltenstern S (2005) Composition of the microbial communities in the mineral soil under different types of natural forest. Soil Biology & Biochemistry 37 (4): 661–671; doi: 10.1016/j.soilbio.2004.08.023
Hernández A, Ascanio MO, Morales DM, Cabrera RA (2005) Correlación de la nueva versión de Clasificación Genética de los Suelos de Cuba con las clasificaciones internacionales y nacionales: una herramienta útil para la investigación, docencia y producción agropecuaria. Instituto Nacional de Investigaciones Agrícolas, La Habana
INS (1999) Clasificación genética de los suelos de Cuba. Instituto Nacional de Suelos MINAG, La Habana
Jenkinson S, Polwlson S (1976) The effects of biocidal treatments on metabolism in soil V A method for measuring soil biomass. Soil Biology and Biochemistry 8 (3): 209-213; doi: 10.1016/0038-0717(76)90005-5
Joergensen R (1996) The fumigation-extraction method to estimate soil microbial biomass: calibration of the kEC value. Soil Biology and Biochemistry 28 (1): 25-31; doi: 10.1016/0038-0717(95)00102-6
John B (2003) Carbon turnover in aggregated soils determined by natural 13C abundance. Ph D dissertation, University of Goettingen, Gottingen, Germany
Kujur M, Patel A K (2014) PLFA Profiling of soil microbial community structure and diversity in different dry tropical ecosystems of Jharkhand. International Journal of Current Microbiology Applied Sciences 3(3): 556-575
McCune N M, Ruiz González Y, Aguila Alcántara E, Fernández Martínez O, Fundora C O, Castillo Arzola N, Cairo Cairo P, D'Haese M, De Neve S, Guevara F (2011) Global questions, local answers: soil management and sustainable intensification in diverse socioeconomic contexts of Cuba. Journal of Sustainable Agriculture 35(6): 650-670; doi: 10.1080/10440046.2011.586595
McKinley P A, White D (2005) Microbial community PLFA and PHB responses to ecosystems restoration in tallgrass prairie soils. Soil Biology and Biochemistry 37 (6): 1946-1958; doi: 10.1016/j.soilbio.2005.02.033
Moeskops B, Sukristiyonubowo BD, Sleutel S, Herawaty L, Husen E, Saraswati R, Setyorini D, De Neve S (2010) Soil microbial communities and activities under intensive organic and conventional vegetable farming in West Java, Indonesia. Applied Soil Ecology 45 (2): 112-120; doi: 10.1016/j.apsoil.2010.03.005
Moore-Kucera J, Dick RP (2008) PLFA Profiling of microbial community structure and seasonal shifts in soils of a Douglas-fir chronosequence. Microb Ecol 55 (3): 500-511; doi: 10.1007/s00248-007-9295-1
ONE (2010) Principales Indicadores del Sector Agropecuario, Anuario Estadístico de Cuba 2008. Oficina Nacional de Estadística, La Habana.
Oyedele A A, Olayungbo A, Denton D, Ogunrewo O, Momodu F O (2015) Assessment of the microbial biomass carbon, nitrogen and phosphorus in relation to physico-chemical properties of Acric Luvisols in Ibadan South West, Nigeria. Journal of Agriculture and Environment for International Development - JAEID 109 (2): 179-187; doi: 10.12895/jaeid.20152.330
Rinklebe J, Langer U (2010) Relationship between soil microbial biomass determined by SIR and PLFA analysis in floodplain soils. Journal of Soils and Sediments 10 (1): 4-8; doi: 10.1007/s11368-009-0155-0
Vance D, Brookes C, Jenkinson S (1987) An extraction method for measuring soil microbial biomass C. Soil Biology and Biochemistry 19 (6): 703-707; doi: 10.1016/0038-0717(87)90052-6
Recibido: 12-01-2016
Aceptado: 25-03-2016
Copyright (c) 2017 Biotecnología Vegetal
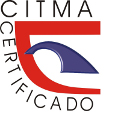
Biotecnología Vegetal eISSN 2074-8647, RNPS: 2154. ISSN 1609-1841, RNPS: 0397 Editada por: Instituto de Biotecnología de las Plantas. Universidad Central Marta Abreu de Las Villas. Carretera a Camajuaní km 5.5, Santa Clara, Villa Clara, Cuba CP 54 830 Tel: 53 42200124, e-mail: info@ibp.co.cu
Biotecnología Vegetal está bajo una Licencia Creative Commons Atribución-NoComercial 4.0 Internacional.