Reseña bibliográfica
Biotecnología Vegetal Vol. 16, No. 4: 195 - 201, octubre - diciembre, 2016
Instituto de Biotecnología de las Plantas. UCLV. MES.
eISSN 2074-8647, RNPS: 2154
General aspects of plant thioredoxins
Aspectos generales de las tioredoxinas en plantas
Milady Francisca Mendoza-Rodríguez
Instituto de Biotecnología de las Plantas, Universidad Central Marta Abreu de Las Villas. Carretera a Camajuaní km 5,5. Santa Clara. Villa Clara. Cuba. CP 54 830. e-mail: milady@ibp.co.cu
ABSTRACT
Chloroplast is the organelle for photosynthesis in eukaryotic cells. In it the presence of a light-dependent ferredoxin/thioredoxin system (FTS) and a NADPH-dependent thioredoxin reductase C (NTRC), have been implicated in plant defense response and at the same time constitute important redox mechanism to provide reducing power to thioredoxins. This organelle also has a critical role in plant innate immunity against pathogen infection, where thioredoxins are the major responsible of to maintain proteins in their reduced state. The present work present a review of the scientific literature about some important aspects of plant thioredoxins.
Keywords: chloroplast, innate immunity, photosynthesis, redox control, stress
RESUMEN
El cloroplasto es el organelo para la fotosíntesis en células eucariotas. En él la presencia del sistema luz-dependiente ferredoxina/tioredoxina (FTS) y de una tioredoxina reductasa C NADPH-dependiente (NTRC), han estado implicados en la respuesta de defensa y al mismo tiempo constituyen importantes mecanismos redox que proveen poder reductor a las tioredoxinas. Este organelo también tiene un papel crítico en la inmunidad innata de las plantas contra la infección de patógenos, donde las tioredoxinas son las principales responsables de mantener las proteínas en su estado reducido. En el presente trabajo se presenta una revisión de la literatura científica acerca de aspectos importantes de las tioredoxinas de plantas.
Palabras clave: cloroplasto, control redox, estrés, fotosíntesis, inmunidad innata
INTRODUCTION
Chloroplasts are the organelle for photosynthesis in eukaryotic cells. Besides, they carry on some other important functions like amino acids, hormones, vitamins, secondary metabolites and lipid biosynthesis, which are components of metabolic pathways and play important roles during stress response and signaling in plant cells .
In this organelle, the presence of a ferredoxin–thioredoxin system (FTS) is linked to photosystem I (PSI) and regulates various chloroplast enzymes. This system together with a NADPH-dependent thioredoxin reductase C (NTRC), provide the reducing power to thioredoxins (TRXs) for their essential role on redox regulation based on disulfide-dithiol conversion of numerous target proteins (Montrichard et al., 2009).
Plants contain several genes encoding TRXs, ubiquitous small oxidoreductases (12 to 14 kDa) which are involved in cell redox regulation. The TRX system in plants is very complex respect to other organisms and based on their primary structure and subcellular localization TRXs have been classified in seven types. The TRXf, TRXm, TRXx, TRXy and TRXz are localized in chloroplasts, TRXo in mitochondria and TRXh mainly in the cytosol. In addition, some TRXh isoforms have also been identified in the apoplast, mitochondria, nuclei and phloem. All of them shared an active site WCG/PPC and a redox-active dithiol CxxC motif (Tovar-Méndez et al., 2011).
TRXs mainly act as electron donors in various metabolic pathways, regulate enzymatic activities and keep the reduced state inside the cell (Montrichard et al., 2009). By other side, a tight control of cellular homeostasis by TRXs is essential for maintenance of normal metabolism and redox-dependent signaling. TRXs are also involved in plant defense response to pathogen infection which has been already seen by several authors (Shim et al., 2004; Campo et al., 2008; Martí et al., 2011). More recently, Lehmann et al. informed that TRXs mediate the activation of the signaling cascade triggered after pathogen recognition.
The chloroplast performs an important role in innate immunity against pathogen attack. However, knowledge about the essential mechanisms involve in regulation of the photosynthetic apparatus and its relationship with the metabolic and regulatory networks of the plant is limited (Tikkanen and Aro, 2014). In this sense, a more comprehensive understanding of this interconnections and the regulatory elements involve in it still awaits future discoveries.
This review is focus on the current knowledge about the role of plant TRXs.
MAIN ROLES OF PLANT THIOREDOXINS
Redox systems
Chloroplast is the factory for photosynthesis in higher plants, a process where the solar energy is converts to chemical energy in organisms such as bacteria and plants. This photosynthetic activity influences the general energy state and redox status by providing NADPH, ATP and carbon skeletons. At the same time, it support the growth of plants and also fuels the initiation and maintenance of responses against external stress factors (Kangasjarvi et al., 2012).
In the chloroplast, FTS is a well-known mechanism that triggers metabolic reactions in response to light-darkness. It comprised ferredoxin (Fd), ferredoxin-thioredoxin reductase (FTR) and TRXf, TRXm, TRXx, TRXy and TRXz. In the presence of light, the photosynthetic electron transfer chain of thylakoid membranes allows reduction of Fd, which can afterward transfer electrons to TRXs through FTR. Then, the reduced TRXs activate target enzymes by reduction of the intramolecular and intermolecular disulfide bridges formed within and between proteins, based on the dynamic changes in the redox potential. Thus, metabolism switches to anabolic pathways (Montrichard et al., 2009).
In such a way, a link is established between the activity of photosynthetic light reactions and the activation of key enzymes of photosynthetic carbon fixation. In this sense, Meyer et at. (2005) from a proteomic approach in Arabidopsis thaliana revealed that 11 enzymes of the Calvin cycle can be regulated by TRXs. Later, Nuruzzaman et al. (2008) informed that both TRXf and TRXm are involved in the regulation of them and other key metabolic enzymes.
The NADP/TRX system is another important redox mechanism that provides reducing power to TRXs that includes NADPH, a TRX and NADPH-dependent TRX reductase (NTR), although chloroplasts contain a modified type designated NTRC. It is widely distributed among organisms and thought to be ubiquitous in aerobes. In this system, the electrons transfer from NADPH to TRXh takes place according to the sequence: NADPH-NTR-TRX-target protein (Montrichard et al., 2009).
In chloroplast, another link is established between photosynthesis and translation. From the photosynthetic machinery, reducing equivalents are transmitted to EF-G (Elongation factor G) via TRXs and that protein synthesis is activated by it in a redox-dependent manner. The elongation of the polypeptide chain is sensible to oxidative stress and their apparent suppression by reactive oxygen species (ROS) could interrupt the redox regulation to prevent further oxidative injury according to Nishiyama et al. (2011).
Besides of the importance of these redox systems in chloroplast functionality some evidences have showed their significance during plant response to stress. In this way, Lim et al. (2010) informed for the first time that silencing of the tomato (Solanum lycopersicum L.) SlFTR-c resulted in spontaneous necrosis development related with higher ROS levels. Moreover, these silenced plants displayed higher resistance to Pseudomonas syringae pv. tomato DC3000 (Pto DC3000) by the activation of defense-related genes. Later on, another authors revealed that the loss-of-function of NTRC resulted in spreading accelerated Pto DC3000 disease-associated cell death with enhanced ROS accumulation in tomato and in A. thaliana (Ishiga et al., 2012). In a similar study plants lacking functional NTRC showed localized cell death (LCD) accompanied by a higher accumulation of hydrogen peroxide (H2O2) in response to nonhost P. syringae pathogens, which suggested the critical role of NTRC in plant innate immunity (Ishiga et al., 2016).
In chloroplast, some important cellular functions are related with the activity of these redox systems. However, just few information support the relevance of them. Based on the current knowledge about FTS system in plants it seems that it works as an important regulator of plant cell death and defense responses but a more detailed biochemical or physiological analyses will be required to confirm it. Besides, a little more emphasis in the study of NADP/TRX system is needed due to their biological role is currently an interesting area to be explored.
Since the discovery of the role of TRXs in the context of photosynthesis, many functions have been adjudged to them. However, some biochemical, molecular and proteomics approaches are still needed to identify putative targets of TRX, which can help to elucidate the main reactions in which they are involved. At the same time, a better understanding of TRX-dependent regulation of several target enzymes is still need it mainly in the thylakoid lumen.
Thioredoxins and oxidative stress
The buildup of ROS is one of the fastest plant defense reactions in response to abiotic and biotic stress. In plants, ROS are formed by the leakage of electrons onto O2 from the electron transport in chloroplasts, mitochondria and plasma membrane or as a byproduct of various metabolic pathways localized in different cellular compartments. The ROS produced after incomplete reduction of oxygen include superoxide anion, H2O2, hydroxyl radical, while singlet oxygen is formed from energy transfer. Among these species, H2O2 acts as signalizing molecule regulating several processes such as cell division, cell wall strengthening, systemic response induction, photosynthesis, phytoalexins production, senescence and stomatal opening (Petrov and Van Breusegem, 2012; Sharma et al., 2012; Lehmann et al., 2015).
Under stress conditions, the balance between the production and elimination of ROS is disturbed. To cope with the detrimental effects of ROS for scavenging or detoxification of them, higher plants have evolved some efficient enzymatic and non-enzymatic antioxidative systems that prevent over-accumulation of redox intermediates and formation of these species. The enzymatic systems include catalase, guaiacol peroxidase, superoxide dismutase and enzymes of ascorbate glutathione cycle. At the same time, ascorbate, carotenoids, glutathione, tocopherols and flavonoids serve as potent non-enzymatic antioxidants within the cell (Aloui et al., 2011; Sharma et al., 2012). Regarding to TRXs, they have been included in the enzymatic antioxidant group where they have the main function of to reduce the disulphide bonds of oxidized proteins.
In plants, the functionality of the antioxidant defenses in the cellular regulation takes place through a complex network involving redox input elements, transmitters, targets and sensory proteins such as TRXs, glutaredoxins and peroxiredoxins (Dietz and Pfannschmidt, 2011).
ROS generation as one of the first plant defense responses after pathogen attack has been associated with disease resistance in plant-fungus interactions, being the hypersensitive response (HR) the most characteristic plant defense reaction (Lehmann et al., 2015). In this context TRXs can act as inhibitors of cell death either directly inhibiting proteins that trigger this process or providing reducing power to proteins that scavenge ROS (Dos Santos and Rey, 2006).
The possible role of Trx in plant disease resistance was informed for the first time by Rivas et al. (2004). These authors founded that a CITRX interacts with the short cytoplasmic tail of Cf-9 and regulates the signaling pathways leading to tomato defense responses against to Cladosporium fulvum Cooke. Furthermore, Laloi et al. (2004) indicated a possible implication of the cytosolic thioredoxin AtTRXh5 in the defense response of A. thaliana to Pto DC3000 and in the oxidative stresses response. Besides, according to Sweat et al. (2007), TRXs are related with plant defense of oats (Avena sativa L.), because its activity is necessary for programmed cell death in response to victorin produced by Cochliobolus victoriae R.R, Nelson.
Another studies carried out from the analysis of cDNA libraries constructed in different pathosystems, based on the polymerase chain reaction-suppression subtractive hybridization (PCR-SSH) (Diatchenko et al., 1996), have offered new evidences about the role of TRXs during host response to pathogen infection. The differential expression of Trx (s) was obtained in rice (Oryza minuta) challenged by Magnaporthe oryzae B.C. Couch (Shim et al., 2004), during early response of ʽCalcutta 4ʼ plants to Mycosphaerella fijiensis Morelet infection (Mendoza-Rodríguez et al., 2006) and in Oryza sativa L. plants at early time points after M. oryzae inoculation (Campo et al., 2008).
The role of mitochondrial thioredoxin PsTRXo1 as a component of the defense system in pea (Pisum sativum L. cv. Lincoln), in providing tolerance against the salt stress was reported by Martí et al. (2011). Recently, Mukaihara et al. (2016) founded a cytosolic TRX, acting as a molecular switch for the activation of effector protein RipAY from Ralstonia solanacearum which degrade GSH, resulting in the suppression of plant pathogen-associated molecular pattern (PAMP)-triggered immunity (PTI) in plants.
Previous information supports the role of TRXs during plant defense response to stress conditions. However, just few studies has been focus on the possible relevance of this gene (s) during host response to pathogen infection.
Now, it is known that the photosynthetic process is essential in plant defense to stress conditions. However, in scientific literature has been made more emphasis in the study of regulation of plant defense responses than in the effects of pathogen infection on metabolic processes such as photosynthesis. For this reason, to elucidate the impact of photosynthesis in the cell reprogramming is still a fundamental question awaiting further molecular and physiological analysis.
Chloroplasts and innate immunity
Chloroplasts have a critical role in plant innate immunity against invading pathogens. Their perception commonly promotes transient increases of ROS generation, secondary messengers that can elicit an HR in infected tissues. According to Kangasjarvi et al. (2014), in plants after an oxidative bursts a transcriptome reprogramming and the induction of disease resistance takes place.
The activation of plant immunity is associated with changes in the cellular redox status, where TRXs are the main responsible of to maintain proteins in their reduced state. This mechanism of redox regulation based on disulfide-dithiol conversion of numerous target proteins, is emerging as a main regulatory system in signal transduction during plant response to biotic and abiotic stress.
Pioneering studies have linked photosynthesis and chloroplast ROS imbalances to plant immunity (Mühlenbock et al., 2008). In addition, it has been informed that the mechanisms controlling photosynthetic light reactions can be involved in plant responses to biotic stress agents. At the same time, chloroplast is the main source of pro-defense molecules, which communicate and coordinate with other organelles during defense, being the H2O2 and salicylic acid (SA) the most significant (Kangasjarvi et al., 2012; Karpinski et al., 2013).
During plant defense response some signals like ROS are produced to control the nuclear gene expression to maintain the cellular homeostasis, or optimizing chloroplast functionality under stress conditions, including pathogen attacks. Low levels of ROS can exert as a retrograde signal, whereas higher levels induce oxidative damage resulting in LCD. Among them H2O2 function as a signaling compound in plant innate immunity (Chi et al., 2015; Hossain et al., 2015).
Previous researches have shown that chloroplast ROS production regulate disease-associated cell death in tomato and Arabidopsis (Ishiga et al., 2009; Ishiga et al., 2012). More recently Caplan et al. (2015) announced the importance of the inter-organellar communication as a vital resource for a successful innate immune response that confer defense against pathogens. At the same time, the relevance of pro-defense molecules in cell-to-cell signaling for stromules induction along the borders of HR-PCD during plant immunity was observed.
An important defense mechanism in plants called systemic acquired resistance (SAR) confers immunity to a broad-spectrum of pathogens and results in accumulation of the signal molecule SA. Changes in gene expression during SAR require SA and is modulated by the interaction between the non expressor of pathogenesis-related genes1 (NPR1) protein, a master regulator of plant immunity and members of the TGA family of transcription factors. This is a very well known mechanism, where under reductive conditions a biphasic change mediated by TRXs converts the inactive oligomeric complex NPR1 to an active monomeric state. Afterwards, this protein is translocated into the nucleus where interacts with the oxidized TGA and induce the defense response genes in plants facing pathogen infection (Lehmann et al., 2015).
Some experimental evidences have shown this relationship. In wheat (Triticum aestivum L.) overexpression of A. thaliana NPR1 gene (AtNPR1) confers resistance to Fusarium head blight (FHB) caused by Fusarium graminearum Schwabe (Makandar et al., 2006). By other side, the overexpression of NPR1 in Arabidopsis and tobacco enhances resistance to the bacterial blight-causing Xanthomonas oryzae pv. oryzae (Yuan et al., 2007).
Even though, a more comprehensive understanding of the underlying mechanisms and regulatory networks imply in plant innate immunity after pathogen challenged have started to emerge, more studies about this theme are still needed to deciphering this complex relationship in different pathosystem.
CONCLUSION
The results presented confirm the advances reached in the understanding of chloroplast functionality and their role during plant response to pathogen infection. However, deeper studies about the role of photosynthesis in plant defense is still a question awaiting further molecular and physiological elucidation, if it is considered that different pathogenesis mechanisms can be trigger by pathogens based on their life cycle.
In spite of the huge knowledge about SA signal generation, regulation and transduction during HR cell death, defense gene expression, and/or SAR the main question concerns about their initial recognizing and how this event triggers resistance signaling. By other side, due to the importance of reductive conditions in the development of an effective plant defense response, a better comprehension of the signaling pathways involve in photosynthesis and detoxification of ROS produced during plant-pathogen interaction is necessary.
REFERENCES
Aloui A, Recorbet G, Robert F, Schoefs B, Bertrand M, Henry C, Gianinazzi-Pearson V, Dumas-Gaudot E, Aschi-Smiti S (2011) Arbuscular mycorrhizal symbiosis elicits shoot proteome changes that are modified during cadmium stress alleviation in Medicago truncatula. BMC Plant Biology 11 (1): 75
Campo S, Manrique S, García-Martínez J, San Segundo B (2008) Production of cecropin A in transgenic rice plants has an impact on host gene expression. Plant Biotechnology Journal 6 (6): 585-608; doi: 10.1111/j.1467-7652.2008.00339.x
Caplan JL, Kumar AS, Park E, Padmanabhan MS, Hoban K, Modla S, Czymmek K, Dinesh-Kumar SP (2015) Chloroplast stromules function during innate immunity. Developmental Cell 34 (1): 45-57; doi: 10.1016/j.devcel.2015.05.011
Chi W, Feng P, Ma J, Zhang L (2015) Metabolites and chloroplast retrograde signaling. Current Opinion in Plant Biology 25: 32-38; doi: 10.1016/j.pbi.2015.04.006
Diatchenko L, Chris Lau Y-F, Campbell AP, Chenchik A, Moqadam F, Huang B, Lukyanov S, Lukyanov K, Gurskaya N, Sverdlov ED, Siebert PD (1996) Suppression subtractive hybridization: a method for generating differentially regulated or tissue-specific cDNA probes and libraries. Proceedings of the National Academy of Sciences USA 93 (12): 6025-6030
Dietz K-J, Pfannschmidt T (2011) Novel regulators in photosynthetic redox control of plant metabolism and gene expression. Plant Physiology 155 (4): 1477-1485
Dos Santos CV, Rey P (2006) Plant thioredoxins are key actors in the oxidative stress response. Trends in Plant Science 11 (7): 329-334
Hossain MA, Bhattacharjee S, Armin S-M, Qian P, Xin W, Li H-Y, Burritt DJ, Fujita M, Tran L-SP (2015) Hydrogen peroxide priming modulates abiotic oxidative stress tolerance: insights from ROS detoxification and scavenging. Frontiers in Plant Science 6: 420; doi: 10.3389/fpls.2015.00420
Ishiga Y, Ishiga T, Wangdi T, Mysore KS, Uppalapati SR (2012) NTRC and chloroplast-generated reactive oxygen species regulate Pseudomonas syringae pv. tomato disease development in tomato and Arabidopsis. Molecular Plant-Microbe Interactions 25 (3): 294-306; doi: 10.1094/ MPMI -05-11-0130
Ishiga Y, Ishiga T, Ikeda Y, Matsuura T, Mysore KS (2016) NADPH-dependent thioredoxin reductase C plays a role in nonhost disease resistance against Pseudomonas syringae pathogens by regulating chloroplast-generated reactive oxygen species. PeerJ 4: e1938; doi: 10.7717/peerj.1938
Ishiga Y, Uppalapati SR, Ishiga T, Elavarthi S, Martin B, Bender CL (2009) Involvement of coronatine-inducible reactive oxygen species in bacterial speck disease of tomato. Plant Signaling and Behavior 4 (3): 237-239; doi: 10.4161/psb.4.3.7915
Kangasjarvi S, Neukermans J, Li S, Aro E-M, Noctor G (2012) Photosynthesis, photorespiration, and light signalling in defence responses. Journal of Experimental Botany 63 (4): 1619-1636; doi: 10.1093/jxb/err402
Kangasjärvi S, Tikkanen M, Durian G, Aro E-M (2014) Photosynthetic light reactions - An adjustable hub in basic production and plant immunity signaling. Plant Physiology and Biochemistry 81: 128-134; doi: 10.1016/j.plaphy.2013.12.004
Karpinski S, Szechynka‐Hebda M, Wituszynska W, Burdiak P (2013) Light acclimation, retrograde signalling, cell death and immune defences in plants. Plant, Cell & Environment 36 (4): 736-744; doi: 10.1111/pce.12018
Laloi C, Mestres-Ortega D, Marco Y, Meyer Y, Reichheld J-P (2004) The Arabidopsis cytosolic thioredoxin h5 gene induction by oxidative stress and its W-box-mediated response to pathogen elicitor. Plant Physiology 134 (3): 1006-1016; doi: 10.1104/pp.103.035782
Lehmann S, Serrano M, L’Haridon F, Tjamos SE, Metraux J-P (2015) Reactive oxygen species and plant resistance to fungal pathogens. Phytochemistry 112: 54-62; doi: 10.1016/j.phytochem.2014.08.027
Lim CJ, Kim WB, Lee B-S, Lee HY, Kwon T-H, Park JM, Kwon S-Y (2010) Silencing of SlFTR-c, the catalytic subunit of ferredoxin:thioredoxin reductase, induces pathogenesis-related genes and pathogen resistance in tomato plants. Biochemical and Biophysical Research Communications 399 (4): 750-754; doi: 10.1016/j.bbrc.2010.08.016
Makandar R, Essig JS, Schapaugh MA, Trick HN, Shah J (2006) Genetically engineered resistance to Fusarium head blight in wheat by expression of Arabidopsis NPR1. Molecular Plant-Microbe Interactions 19 (2): 123-129; doi: 10.1094/MPMI-19-0123
Martí MC, Florez-Sarasa I, Camejo D, Ribas-Carbó M, Lázaro JJ, Sevilla F, Jiménez A (2011) Response of mitochondrial thioredoxin PsTrxo1, antioxidant enzymes, and respiration to salinity in pea (Pisum sativum L.) leaves. Journal of Experimental Botany 61 (11): 3863-3874; doi: 10.1093/jxb/err076
Mendoza-Rodríguez MF, Sánchez-Rodríguez A, Acosta-Suárez M, Roque B, Portal O, Jiménez E (2006) Construcción y secuenciación parcial de una biblioteca sustractiva en ‘Calcutta 4' (Musa AA) en estadio temprano de infección con Mycosphaerella fijiensis Morelet. Biotecnología Vegetal 6 (4): 213-217
Meyer Y, Reichheld JP, Vignols F (2005) Thioredoxins in Arabidopsis and other plants. Photosynthesis Research 86 (3): 419-433
Montrichard F, Alkhalfioui F, Yano H, Vensel WH, Hurkman WJ, Buchanan BB (2009) Thioredoxin targets in plants: The first 30 years. Journal of Proteomics 72 (3): 452-474; doi: 10.1016/j.jprot.2008.12.002
Mühlenbock P, Szechyńska-Hebda M, Płaszczyca M, Baudo M, Mateo A, Mullineaux PM, Parker JE, Karpińska B, Karpiński S (2008) Chloroplast signaling and LESION SIMULATING DISEASE1 regulate crosstalk between light acclimation and immunity in Arabidopsis. The Plant Cell 20 (9): 2339-2356
Mukaihara T, Hatanaka T, Nakano M, Oda K (2016) Ralstonia solanacearum Type III effector RipAY is a glutathione-degrading enzyme that is activated by plant cytosolic thioredoxins and suppresses plant immunity. mBio 7 (2): e00359-00316; doi: 10.1128/mBio.00359-16
Nishiyama Y, Allakhverdiev SI, Murata N (2011) Protein synthesis is the primary target of reactive oxygen species in the photoinhibition of photosystem II. Physiologia Plantarum 142 (1): 35-46; doi: 10.1111/j.1399-3054.2011.01457.x
Nuruzzaman M, Gupta M, Zhang C, Wang L, Xie W, Xiong L, Zhang Q, Lian X (2008) Sequence and expression analysis of the thioredoxin protein gene family in rice. Molecular Genetics and Genomics 280 (2): 139-151; doi: 10.1007/s00438-008-0351-4
Petrov VD, Van Breusegem F (2012) Hydrogen peroxide-a central hub for information flow in plant cells. AoB PLANTS 2012: pls014; doi: 10.1093/aobpla/pls014
Rivas S, Rougon-Cardoso A, Smoker M, Schauser L, Yoshioka H, Jones JD (2004) CITRX thioredoxin interacts with the tomato Cf-9 resistance protein and negatively regulates defence. The EMBO Journal 23 (10): 2156-2165; doi: 10.1038/sj.emboj.7600224
Rolland N, Curien G, Finazzi G, Kuntz M, Maréchal E, Matringe M, Ravanel S, Seigneurin-Berny D (2012) The biosynthetic capacities of the plastids and integration between cytoplasmic and chloroplast processes. Annual Review of Genetics 46: 233-264; doi: 10.1146/annurev-genet-110410-132544
Seyfferth C, Tsuda K (2014) Salicylic acid signal transduction: the initiation of biosynthesis, perception and transcriptional reprogramming. Frontiers in Plant Science 5: 697; doi: 10.3389/fpls.2014.00697
Sharma P, Jha AB, Dubey RS, Pessarakli M (2012) Reactive oxygen species, oxidative damage, and antioxidative defense mechanism in plants under stressful conditions. Journal of Botany 2012; doi: 10.1155/2012/217037
Shim KS, Cho SK, Jeung JU, Jung KW, You MK, Ok SH, Chung YS, Kang KH, Hwang HG, Choi HC, Moon HP, Shin JS (2004) Identification of fungal (Magnaporthe grisea) stress-induced genes in wild rice (Oryza minuta). Plant Cell Reports 22 (8): 599-607; doi: 10.1007/s00299-003-0742-2
Sweat TA, Wolpert TJ (2007) Thioredoxin h5 is required for victorin sensitivity mediated by a CC-NBS-LRR gene in Arabidopsis. The Plant Cell 19 (2): 673-687; doi: 10.1105/tpc.106.047563
Tikkanen M, Aro E-M (2014) Integrative regulatory network of plant thylakoid energy transduction. Trends in Plant Science 19 (1): 10-17; doi: 10.1016/j.tplants.2013.09.003
Tovar-Méndez A, Matamoros MA, Bustos-Sanmamed P, Dietz K-J, Cejudo FJ, Rouhier N, Sato S, Tabata S, Becana M (2011) Peroxiredoxins and NADPH-dependent thioredoxin systems in the model legume Lotus japonicus. Plant Physiology 156 (3): 1535-1547; doi: 10.1104/pp.111.177196
Yuan Y, Zhong S, Li Q, Zhu Z, Lou Y, Wang L, Wang J, Wang M, Li Q, Yang D, He Z (2007) Functional analysis of rice NPR1-like genes reveals that OsNPR1/NH1 is the rice orthologue conferring disease resistance with enhanced herbivore susceptibility. Plant Biotechnology Journal 5 (2): 313-324; doi: 10.1111/j.1467-7652.2007.00243.x
Recibido: 06-06-2016
Aceptado: 01-09-2016
Copyright (c) 2017 Biotecnología Vegetal
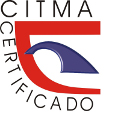
Biotecnología Vegetal eISSN 2074-8647, RNPS: 2154. ISSN 1609-1841, RNPS: 0397 Editada por: Instituto de Biotecnología de las Plantas. Universidad Central Marta Abreu de Las Villas. Carretera a Camajuaní km 5.5, Santa Clara, Villa Clara, Cuba CP 54 830 Tel: 53 42200124, e-mail: info@ibp.co.cu
Biotecnología Vegetal está bajo una Licencia Creative Commons Atribución-NoComercial 4.0 Internacional.